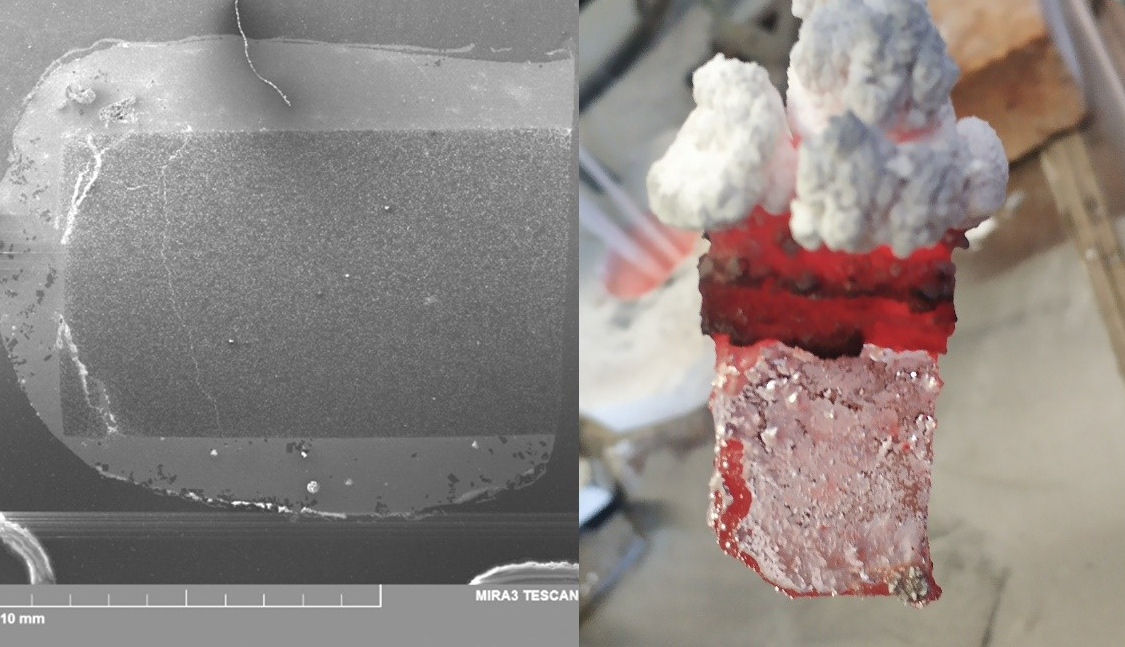
Borated graphite cathodes for low-temperature aluminum electrolysis
Abstract
Electrochemical boriding of the graphite plates in the potassium cryolite based electrolytes was studied. The boriding were carried out in a cell with vertical electrodes. The procedure included 2 stages: 1) electrolysis in the KF–AlF3–KBF4 melt (CR=1.3) at low current density (0.01–0.02 A/cm2), required for the boron reduction, at 700 and 750 °C; 2) electrolysis in the KF–AlF3–Al2O3 melt at higher current density (0.2 A/cm2), required for the aluminum reduction. The optimal conditions of electrodeposition for obtaining the borated wettable cathodes were determined. According to the SEM data, a continuous AlB2 layer with a thickness of 7–10 μm was formed on the graphite surface. The borated graphite was tested as a wetted cathode during the low-temperature aluminum electrolysis. Prolonged electrolysis in a vertical cell with the graphite anode and the borated graphite cathode was carried out in the KF–NaF(10 wt.%)–AlF3–Al2O3 electrolyte (CR=1.5) at 830 °C. After 100 h of electrolysis, the thickness of the AlB2 layer on the graphite surface was 5 μm, while the cathode surface was completely wetted with aluminum. Thus, we demonstrated the feasibility of using the borated graphite cathode as a wettable dripping cathode in the low-temperature aluminum electrolysis in the vertical cell.
Keywords
Full Text:
PDFReferences
Haupin WE. Principles of aluminum electrolysis. Essent Read Light Met. 2016;2(3):3–11. doi:10.1007/978-3-319-48156-2_1
Evans J, Kvande H. Sustainability, climate change, and greenhouse gas emissions reduction: Responsibility, key challenges, and opportunities for the aluminum industry. JOM. 2008;60(8):25–31. doi:10.1007/s11837-008-0103-2
Leber BP. Perfluorocarbon (PFC) generation at primary aluminum smelters. Essent Read Light Met. 2016;2:1015–1023. doi:10.1007/978-3-319-48156-2_150
Galasiu I, Galasiu R, Thonstad,J, Inert anodes for alumini-um electrolysis. Aluminium-Verlag. 2007. 207 p.
Thonstad J. The solubility of aluminium in NaF–AlF3–Al2O3 melts. Can J Chem. 1965;43:3429. doi:10.1139/v65-476
Kubinakova E, Danielik V, Hıves J. Electrochemical characterization of low-temperature molten mixture systems suitable as an innovation in aluminum technology. J Elec-trochem Soc. 2018;165(14):274. doi:10.1016/j.electacta.2018.01.174
Redkin A, Apisarov A, Dedyukhin A, Kovrov V, Zaikov Yu, Tkacheva O, Hryn J. Recent developments in low-temperature electrolysis of aluminum. ECS Trans. 2012;50(11):205–213. doi:10.1016/j.molliq.2017.02.021
Apisarov A, Dedyukhin A, Red'kin A, Tkacheva O, Zaikov Yu. Physicochemical properties of KF-NaF-AlF3 molten electrolytes. Russ J Electrochem. 2010;46(6):633–639. doi:10.1134/S1023193510060066
Beck TR, MacRAE CM, Wilson NC. Metal anode performance in low-temperature electrolytes for aluminum production. Met Mater Trans B. 2011;42(4):807–813. doi:10.1007/s11663-011-9511-8
Tkacheva O, Hryn J, Spangenberger J, Davis B, Alcorn T. Operating Parameters of Aluminum Electrolysis in a KF-AlF3 based Electrolyte. Light Met. 2012;675–680. doi:10.1007/978-3-319-48179-1_116
Hryn J, Tkacheva O, Spangenberger J. Initial 1000A aluminum electrolysis testing in potassium cryolite-based electrolyte. Light Met. 2016;1289–1294. doi:10.1007/978-3-319-65136-1_217
Zaikov Yu, Khramov A, Kovrov V, Kryukovsky V, Apisarov A, Chemesov O, Shurov N, Tkacheva O. Electrolysis of aluminum in the low melting electrolytes based on potassium cryolite. Light Met. 2009;505.
Cui P, Solheim A, Haarberg GM. The performance of aluminium electrolysis in a low temperature electrolyte system. Light Met. 2016;383–387. doi:10.1007/978-3-319-48251-4_63
Yasinskiy AS, Padamata SK, Polyakov PV, Shabanov AV. An update on inert anodes for aluminium electrolysis. Non-ferrous Met.2020;48(1):15–23. doi:10.17580/nfm.2020.01.03
Helle S, Brodu B, Davis B, Guay D, Roue L. Influence of the iron content in Cu–Ni based inert anodes on their corrosion resistance for aluminium electrolysis. Corros Sci. 2011;53(11):3248–3253. doi:10.1016/j.corsci.2011.05.069
Nguyen T, de Nora V. De Nora oxygen evolving inert metallic anode. Light Met. 2006;385–390.
Glucina M, Hyland M, Laboratory-scale performance of a binary Cu–Al alloy as an anode for aluminium electrowinning. Corros Sci. 2006.48(9):2457–2469. doi:10.1016/j.corsci.2005.09.013
Bradford DR. Inert anode metal life in low temperature reduction process. Nat Tech Rep Lib. 2005;101. Available from: https://ntrl.ntis.gov/NTRL/dashboard/searchResults/titleDetail/DE2006841153.xhtml
Suzdaltsev AV, Nikolaev AYu, Zaikov YuP. Towards the stability of low-temperature aluminum electrolysis. J Electro-chem Soc. 2021;168(4);046521. doi:10.1149/1945-7111/abf87f
Wang J, Lai Y, Tian Z, Liu Y. Effect of electrolysis superheat degree on anticorrosion performance of 5Cu/(10NiO-NiFe2O4) cermet inert anode. J Cent South Univ Technol. 2007;14(6):768–772. doi:10.1007/s11771-007-0146-5
Nikolaev AYu, Suzdaltsev AV, Polyakov PV, Zaikov YuP. Cathode process at the electrolysis of KF-AlF3-Al2O3 melts and suspensions. J Electrochem Soc. 2017;164(8):5315–5321. doi:10.1149/2.0491708jes
Pawlek RP. Wettable cathodes: an update. Essen Read Light Met. 2010;4;1185–1190. doi:10.1007/978-3-319-48200-2_157
Brown CV. Final Report: Wetted Cathodes for Low-Temperature Aluminum Smelting. Mater Sci. 2002;51. doi:10.2172/801562
Rybakova N, Souto M, Martinz HP, Andriyko Y, Artner W, Godinho J, Nauer GE. Stability of electroplated titanium diboride coatings in high-temperature corrosive media. Corros Sci. 2009;51(6):1315–1321. doi:10.1016/j.corsci.2009.03.020
Rybakova N, Souto M, Martinz HP, Andriyko Y, Artner W, Godinho J, Nauer GE. Morphology and mechanical properties of tib2 coatings deposited from chloride-fluoride melts by pulse electroplating. J Electrochem Soc. 2009;156(4):131. doi:10.1149/1.3060508
Sista V, Kahvecioglu O, Kartal G, Zeng QZ, Kim JH, Eryilmaz OL, Erdemir A. Evaluation of electrochemical bonding of Inconel 600. Surf Coat Technol. 2013;215:452–549. doi:10.1016/j.surfcoat.2012.08.083
Kartal G, Eryilmaz OL, Krumdick G, Erdemir A, Timur S. Kinetics of electrochemical boriding of low carbon steel. Appl Surf Sci. 2011;257(15):6928–6934. doi:10.1016/j.apsusc.2011.03.034
Polyakova LP, Bukatova GA, Polyakova EG, Christensen E, Barner JH, Bjerrum N. Electrochemical behavior of boron in LiF‐NaF‐KF‐ melts. J Electrochem Soc. 1996;143(10):3178–3191. doi:10.1149/1.1837184
Raj SC, Skyllas-KIazacos M. Electrochemical studies of the effect of tio2 and b2o3 additions on the aluminum deposition reaction in the molten cryolite bath. Electrochim Acta. 1992;37(10):17871796. doi:10.1016/0013-4686(92)85082-V
Kataev AA, Karimov KR, Chernov YaB, Kulik NP, Malkov VB, Antonov BD, Vovkotrub EG, Zaikov YuP. Wetting of boride cathode coatings by low-melting-point cryolite and liquid aluminum. Rus Met. 2010;8:689–701. doi:10.1134/S0036029510080057
Kataev A, Tkacheva O, Molchanova N, ZaikovYu. Production of the Al–B Master alloy by aluminothermal reduction of KBF4 and B2O3 in a medium of the molten salt flux. Russ J Non-Ferrous Met. 2019;60(4):354–362. doi:10.3103/S1067821219040059
Chrenkova M, Danek V, Vasiljev R, Silny A, Kremenetsky V,Polyakov E. Density and viscosity of the (LiF–NaF–KF)(eut)–KBF4–B2O3 melts. J Mol Liq. 2003;102(1–3):213–226. doi:10.1016/S0167-7322(02)00063-6
Kataev AA, Rudenko AV, Reznitskikh OG, Molchanova NG, Dedyukhin AE, Redkin AA, Zaikov OYu. Thermal stability of KBF4 in fusible cryolite melts. Rasplavy. 2014;6(12):12–17.
Nikolaev A, Suzdaltsev A, Zaikov Yu. Cathode process in the KF-AlF3-Al2O3 melts. J Electrochem Soc. 2019;166(15):784–791. doi:10.1149/2.0521915jes
Keniry J. The economics of inert anodes and wettable cathodes for aluminum reduction cells. JOM. 2001;5:43–47. doi:10.1007/s11837-001-0209-2
Filatov AA, Suzdaltsev AV, Zaikov YuP. Cathode processes in the KF–AlF3–Al2O3–B2O3 melt. Rasplavy. 2022;1:61–72. doi:10.31857/S0235010622010030
Kataev AA, Tkacheva OYu, Molchanova NG, Zaikov YuP. Production of the Al–B Master alloy by aluminothermal reduction of KBF4 and B2O3 in a medium of the molten salt flux. Russ J Non-Ferrous Met.2019;60(4),354–362. doi:10.3103/S1067821219040059
Wang X. Boride phase formation in the production of Al-B master alloys. J Alloys Compd. 2017;722:302–306. doi:10.1016/j.jallcom.2017.06.107
DOI: https://doi.org/10.15826/chimtech.2022.9.2.08
Copyright (c) 2022 Rudenko A.V., Kataev A.A., Neupokoeva M.M., Tkacheva O.Yu.

This work is licensed under a Creative Commons Attribution 4.0 International License.
Chimica Techno Acta, 2014–2025
eISSN 2411-1414
Copyright Notice