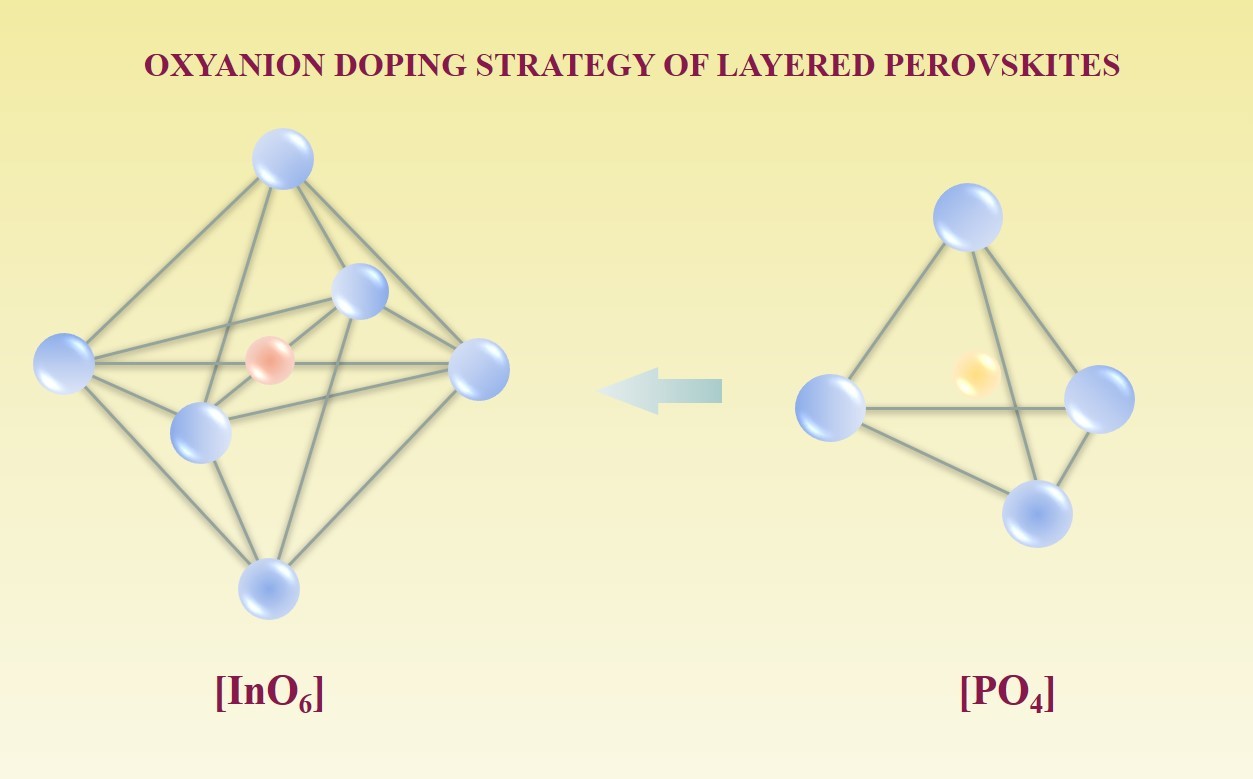
Phosphorus-doped protonic conductors based on BaLanInnO3n+1 (n = 1, 2): applying oxyanion doping strategy to the layered perovskite structure
Abstract
Keywords
Full Text:
PDFReferences
Panwar NL, Kaishik SC, Kothari S. Winkler T, Sass FA, Duda GN, Schmidt-Bleek K. Role of renewable energy sources in environmental protection: A review. Renew Sustain Energy Rev. 2011;15(3):1513–1524. doi:10.1016/j.rser.2010.11.037
Chu S, Majumdar A. Opportunities and challenges for a sustainable energy future. Nature. 2012;488:294–303. doi:10.1038/nature11475
Dincer I, Rosen MA. Sustainability aspects of hydrogen and fuel cell systems. Int J Sustain Energy Dev. 2011;15(2):137–146. doi:10.1016/j.esd.2011.03.006
Branco H, Castro R, Lopes AS. Battery energy storage systems as a way to integrate renewable energy in small iso-lated power systems. Int J Sustain Energy Dev. 2018;43:90–99. doi:10.1016/j.esd.2018.01.003
Malerba D. Poverty-energy-emissions pathways: Recent trends and future sustainable development goals. Int J Sus-tain Energy Dev. 2019;49:109–124. doi:10.1016/j.esd.2019.02.001
Buonomano A, Barone G, Forzano C. Advanced energy technologies, methods, and policies to support the sustainable development of energy, water and environment systems. Energy Rep. 2022;8:4844–4853. doi:10.1016/j.egyr.2022.03.171
Olabi AG, Abdelkareem MA. Renewable energy and climate change. Renew Sustain Energy Rev. 2022;158:112111. doi:10.1016/j.rser.2022.112111
Østergaard PA, Duic N, Noorollahi Y, Mikulcic H, Kalogirou S. Sustainable development using renewable energy technology. Renew Energy. 2020;146:2430–2437. doi:10.1016/j.renene.2019.08.094
International Energy Agency. The Future of Hydrogen: Seizing today’s opportunities. OECD. 2019. doi:10.1787/1e0514c4-en
Abdalla AM, Hossain S, Nisfindy OB, Azad AT, Dawood M, Azad AK. Hydrogen production, storage, transportation and key challenges with applications: A review. Energy Convers Manag. 2018;165:602–627. doi:10.1016/j.enconman.2018.03.088
Dawood F, Anda M, Shafiullah GM. Hydrogen production for energy: An overview. Int J Hydrog Energy. 2020;45(7):3847–3869. doi:10.1016/j.ijhydene.2019.12.059
Arsad AZ, Hannan MA, Al-Shetwi AQ, Mansur M, Muttaqi KM, Dong ZY, Blaabjerg F. Hydrogen energy storage integrated hybrid renewable energy systems: A review analysis for future research directions. Int J Hydrog Energy. 2022;47(39):17285–17312. doi:10.1016/j.ijhydene.2022.03.208
Hossain S, Abdalla AM, Jamain SNB, Zaini JH, Azad AK. A review on proton conducting electrolytes for clean energy and intermediate temperature-solid oxide fuel cells. Renew Sustain Energy Rev. 2017;79:750–764. doi:10.1016/j.rser.2017.05.147
Kim J, Sengodan S, Kim S, Kwon O, Bu Y, Kim G. Proton conducting oxides: A review of materials and applications for renewable energy conversion and storage. Renew Sustain Energy Rev. 2019;109:606–618. doi:10.1016/j.rser.2019.04.042
Zhang W, Hu YH. Progress in proton-conducting oxides as electrolytes for low-temperature solid oxide fuel cells: From materials to devices. Energy Sci Eng. 2021;9(7):984–1011. doi:10.1002/ese3.886
Meng Y, Gao J, Zhao Z, Amoroso J, Tong J, Brinkman KS. Review: recent progress in low-temperature proton-conducting ceramics. J Mater Sci. 2019;54:9291–9312. doi:10.1007/s10853-019-03559-9
Medvedev D. Trends in research and development of protonic ceramic electrolysis cells. Int J Hydrog Energy. 2019;44(49):26711–26740. doi:10.1016/j.ijhydene.2019.08.130
Medvedev DA. Current drawbacks of proton-conducting ceramic materials: How to overcome them for real electro-chemical purposes. Curr Opin Green Sustain Chem. 2021;32:100549. doi:10.1016/j.cogsc.2021.100549
Zvonareva I, Fu X-Z, Medvedev D, Zhao Z. Electrochemistry and energy conversion features of protonic ceramic cells with mixed ionic-electronic electrolytes. Energy Environ Sci. 2022;15:439–465. doi:10.1039/D1EE03109K
Shim JH. Ceramics breakthrough. Nature Energy. 2018;3:168–169. doi:10.1038/s41560-018-0110-7
Bello IT, Zhai S, He Q, Cheng C, Dai Y, Chen B, Zhang Y, Ni M. Materials development and prospective for protonic ceramic fuel cells. Int J Energy Res. 2021;46(3):2212–2240. doi:10.1002/er.7371
Irvine J et al. Roadmap on inorganic perovskites for energy applications. J Phys Energy. 2021;3:031502. doi:10.1088/2515-7655/abff18
Chiara A, Giannici F, Pipitone C, Longo A, Aliotta M, Gam-bino M, Martorana A. Solid-Solid Interfaces in Protonic Ceramic Devices: A Critical Review. ACS Appl Mater Interfaces. 2020;12:55537–55553. doi:10.1021/acsami.0c13092
Cao J, Ji Y, Shao Z. New Insights into the Proton-Conducting Solid Oxide Fuel Cells. J Chin Ceram Soc. 2021;49:83–92. doi:10.14062/j.issn.0454-5648.20200390
Bello IT, Zhai S, Zhao S, Li Z, Yu N, Ni M. Scientometric review of proton-conducting solid oxide fuel cells. Int J Hydrog Energy. 2021;46(75):37406–37428. doi:10.1016/j.ijhydene.2021.09.061
Iwahara H, Esaka T, Uchida H, Maeda N. Proton conduction in sintered oxides and its application to steam electrolysis for hydrogen production. Solid State Ion. 1981;3–4:359–363. doi:10.1016/0167-2738(81)90113-2
Iwahara H, Uchida H, Maeda N. High temperature fuel and steam electrolysis cells using proton conductive solid electrolytes. J Power Sources. 1982;7(3):293–301. doi:10.1016/0378-7753(82)80018-9
Tarasova N, Colomban P, Animitsa I. The short-range structure and hydration process of fluorine-substituted double perovskites based on barium-calcium niobate Ba2CaNbO5.5. J Phys Chem Solids. 2018;118:32–39. doi:10.1016/j.jpcs.2018.02.049
Fop S, McCombie KS, Wildman EJ, Skakle MS, Irvine JTS, Connor PA, Savaniu C, Ritter C, Mclaughlin AC. High oxide ion and proton conductivity in a disordered hexagonal perovskite. Nature Mater. 2020;19:752–757. doi:10.1038/s41563-020-0629-4
Yashima M, Tsujiguchi T, Sakuda Y, Yasui Y, Zhou Y, Fujii K, Torii S, Kamiyama T, Skinner SJ. High oxide-ion conductivity through the interstitial oxygen site in Ba7Nb4MoO20-based hexagonal perovskite related oxides. Nature Comm. 2021;12:556. doi:10.1038/s41467-020-20859-w
Wang Y, Wang H, Liu T, Chen F, Xia C. Improving the chemical stability of BaCe0.8Sm0.2O3−δ electrolyte by Cl doping for proton-conducting solid oxide fuel cell. Electrochem Comm. 2013;28:87–90. doi:10.1016/j.elecom.2012.12.012
Zhou H, Dai L, Jia L, Zhu J, Li Y, Wang L. Effect of fluorine, chlorine and bromine doping on the properties of gadolinium doped barium cerate electrolytes. Int J Hydrog Energy. 2015; 40(29):8980–8988. doi:10.1016/j.ijhydene.2015.05.040
Tarasova N, Animitsa I. The influence of anionic heterovalent doping on transport properties and chemical stability of F-, Cl-doped brownmillerite Ba2In2O5. J Alloys Compd. 2018;739:353–359. doi:10.1016/j.jallcom.2017.12.317
Ushakov AE, Merkulov OV, Markov AA, Patrakeev MV, Leonidov IA. Ceramic and transport properties of halogen-substituted strontium ferrite. Ceram Int. 2018;44(10):11301–11306. doi:10.1016/j.ceramint.2018.03.177
Liu J, Jin Z, Miao L, Ding J, Tang H, Gong Z, Peng R, Liu W. A novel anions and cations co-doped strategy for developing high-performance cobalt-free cathode for intermediate-temperature proton-conducting solid oxide fuel cells. Int J Hydrog Energy. 2019;44(21):11079–11087. doi:10.1016/j.ijhydene.2019.03.001
Shin JF, Orera A, Apperley DC, Slater PR. Oxyanion doping strategies to enhance the ionic conductivity in Ba2In2O5. J Mater Chem. 2011;21(3):874–879. doi:10.1039/c0jm01978j
Hancock CA, Porras-Vazquez JM, Keenan PJ, Slater PR. Oxy-anions in perovskites: from superconductors to solid oxide fuel cells. Dalton Trans. 2015;44(23):10559. doi:10.1039/c4dt03036b
Tarasova N, Animitsa I. AIILnInO4 with Ruddlesden-Popper structure for electrochemical applications: relationship between ion (oxygen-ion, proton) conductivity, water uptake and structural changes. Materials. 2022;15(1):114. doi:10.3390/ma15010114
Fujii K, Shiraiwa M, Esaki Y, Yashima M, Hoshikawa A, Ishigaki T, Hester JR. New Perovskite-Related Structure Family of Oxide-Ion Conducting Materials NdBaInO4. Chem Mater. 2014;26(8):2488–2491. doi:10.1021/cm500776x
Fujii K, Shiraiwa M, Esaki Y, Yashima M, Kim SJ, Lee S. Improved oxide-ion conductivity of NdBaInO4 by Sr doping. J Mater Chem A. 2015;3(22):11985–11990. doi:10.1039/c5ta01336d
Ishihara T, Yan Y, Sakai T, Ida S. Oxide ion conductivity in doped NdBaInO4. Solid State Ion. 2016;288:262–265. doi:10.1016/j.ssi.2016.01.011
Yang X, Liu S, Lu F, Xu J, Kuang X. Acceptor Doping and Oxygen Vacancy Migration in Layered Perovskite NdBaInO4 Based Mixed Conductors. J Phys Chem C, 2016;12:6416–6426. doi:10.1021/acs.jpcc.6b00700
Fujii K, Yashima M. Discovery and development of BaNdInO4 - A brief review. J Ceram Soc Japan. 2018;126(10):852–859. doi:10.2109/jcersj2.18110
Zhou Y, Shiraiwa M, Nagao M, Fujii K, Tanaka I, Yashima M, Baque L, Basbus JF, Mogni LV, Skinner SJ. Protonic Conduction in the BaNdInO4 Structure Achieved by Acceptor Doping. Chem Mater. 2021;33(6):2139–2146. doi:10.1021/acs.chemmater.0c04828
Kato S, Ogasawara M, Sugai M, Nakata S. Synthesis and oxide ion conductivity of new layered perovskite La1-xSr1+xInO4-d. Solid State Ion. 2002;149(1–2):53–57. doi:10.1016/S0167-2738(02)00138-8
Troncoso L, Alonso JA, Aguadero A. Low activation energies for interstitial oxygen conduction in the layered perov-skites La1+xSr1-xInO4+d. J Mater Chem A. 2015;3(34):17797–17803. doi:10.1039/c5ta03185k
Troncoso L, Alonso JA, Fernández-Díaz MT, Aguadero A. Introduction of interstitial oxygen atoms in the layered perovskite LaSrIn1−xBxO4+δ system (B=Zr, Ti). Solid State Ionics. 2015;282:82–87. doi:10.1016/j.ssi.2015.09.014
Troncoso L, Mariño C, Arce MD, Alonso JA. Dual oxygen defects in layered La1.2Sr0.8-xBaxInO4+d (x = 0.2, 0.3) oxide-ion conductors: a neutron diffraction study. Mater. 2019;12(10):1624. doi:10.3390/ma12101624
Troncoso L, Arce MD, Fernández-Díaz MT, Mogni LV, Alonso JA. Water insertion and combined interstitial-vacancy oxy-gen conduction in the layered perovskites La1.2Sr0.8-xBaxInO4+d. New J Chem. 2019;43(15):6087–6094. doi:10.1039/C8NJ05320K
Tarasova N, Galisheva A, Animitsa I, Korona D, Kreimesh H, Fedorova I. Protonic Transport in Layered Perovskites BaLanInnO3n+1 (n = 1, 2) with Ruddlesden-Popper Structure. Appl Sci. 2022;12(8):4082. doi:10.3390/app12084082
Tarasova N, Animitsa I, Galisheva A. Electrical properties of new protonic conductors Ba1+хLa1–хInO4–0.5х with Ruddlesden-Popper structure. J Solid State Electrochem. 2020;24:1497–1508. doi:10.1007/s10008-020-04630-1
Tarasova N, Galisheva A, Animitsa I. Improvement of oxygen-ionic and protonic conductivity of BaLaInO4 through Ti doping. Ionics. 2020;26:5075–5088. doi:10.1007/s11581-020-03659-6
Tarasova N, Galisheva A, Animitsa I. Ba2+/Ti4+- codoped layered perovskite BаLaInO4: the structure and ionic (O2−, H+) conductivity. Int J Hydrog Energy. 2021;46(32):16868–16877. doi:10.1016/j.ijhydene.2021.02.044
Tarasova NA, Galisheva AO, Animitsa IE, Lebedeva EL. Oxy-gen-Ion and Proton Transport in Sc-Doped Layered Perovskite BaLaInO4. Russ J Electrochem. 2021;57(10):1008–1014. doi:10.1134/S1023193521080127
Tarasova N, Galisheva A, Animitsa I, Anokhina I, Gilev P, Cheremisina P. Novel mid-temperature Y3+ → In3+ doped proton conductors based on the layered perovskite BaLaInO4. Ceram Int. 2022;48(11):15677–15685. doi:10.1016/j.ceramint.2022.02.102
Tarasova N, Galisheva A, Animitsa I, Korona D, Davletbaev K. Novel proton-conducting layered perovskite based on BaLaInO4 with two different cations in B-sublattice: Synthesis, hydration, ionic (O2+, H+) conductivity. Int J Hydrog Energy. 2022;47(44):18972–18982. doi:10.1016/j.ijhydene.2022.04.112
Tarutin A, Lyagaeva J, Medvedev D, Bi L, Yaremchenko A. Recent advances in layered Ln2NiO4+δ nickelates: funda-mentals and prospects of their applications in protonic ceramic fuel and electrolysis cells. J Mater Chem A. 2021;9(1):154–195. doi:10.1039/D0TA08132A
Tarutin A, Gorshkov Yu, Bainov A, Vdovin G, Vylkov A, Lya-gaeva J, Medvedev D. Barium-doped nickelates Nd2–xBaxNiO4+δ as promising electrode materials for protonic ceramic electrochemical cells. Ceram Int. 2020;46(15):24355–24364. doi:10.1016/j.ceramint.2020.06.217
Tarutin A, Lyagaeva J, Farlenkov A, Plaksin S, Vdovin G, Demin A, Medvedev D. A Reversible protonic ceramic cell with symmetrically designed Pr2NiO4+δ-based electrodes: fabrication and electrochemical features. Mater. 2019;12(1):118. doi:10.3390/ma12010118
Tarutin AP, Lyagaeva JG, Farlenkov AS, Vylkov AI, Medvedev DA. Cu-substituted La2NiO4+δ as oxygen electrodes for protonic ceramic electrochemical cells. Ceramic Int. 2019;45(13): 16105–16112. doi:10.1016/j.ceramint.2019.05.127
Shannon RD, Revised effective ionic radii and systematic studies of interatomic distances in halides and chalcogenides. Acta Cryst. 1976;A32:751–767. doi:10.1107/S0567739476001551
DOI: https://doi.org/10.15826/chimtech.2022.9.4.05
Copyright (c) 2022 Nataliia Tarasova, Anzhelika Galisheva

This work is licensed under a Creative Commons Attribution 4.0 International License.
© Website Chimica Techno Acta, 2014–2024
ISSN 2411-1414 (Online)
This journal is licensed under a Creative Commons Attribution 4.0 International