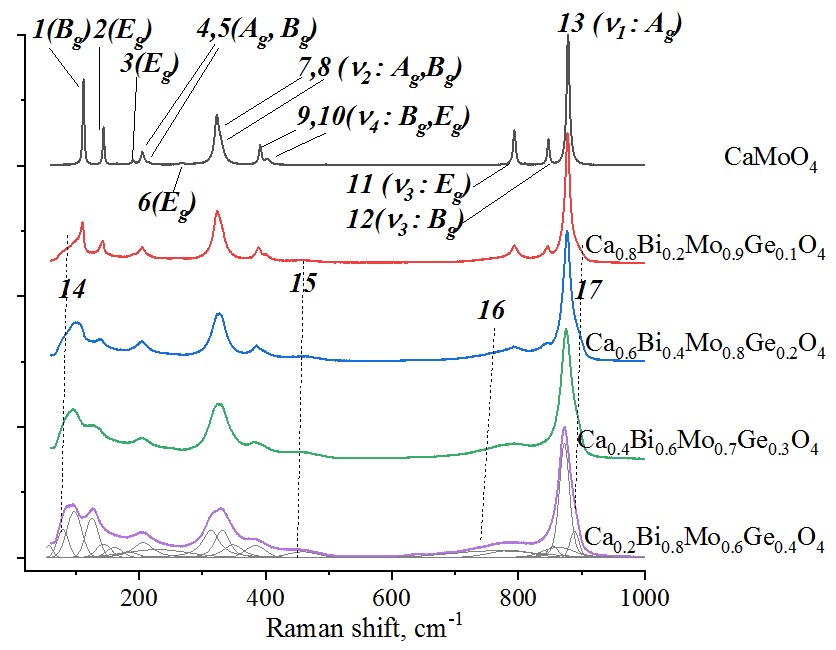
Structure and microwave dielectric properties of Bi- and Ge-doped calcium molybdate
Abstract
The powders of Ca1–2xBi2xMo1–xGexO4 solid solutions weresynthesized by the conventional solid state method and investigated by X–ray powder diffraction and Raman spectroscopy. The Ca1–2xBi2xMo1–xGexO4 compositions crystallize in scheelite structure (sp. gr. I41/a) at 0 <x < 0.4, but traces of bismuth germanates are detected by scanning electron microscopy. The x = 0.5 composition contains several phases with BiVO4- type structures. Raman spectroscopy detected the deformation of [BO4]2– polyhedra and changes in B–O bond length (B = Mo, Ge). The energy gaps were calculated from the diffuse scattering spectra by the Kubelka-Munk method. Energy gap (Eg) values decrease with x from 3.29 eV to 2.91 eV, probably due to 6s2 electrons of bismuth in the valence band and changes of the conductivity band by electrons of germanium. Such values of Eg can provide photocatalytical activity of powders under UV and visible light. The electrodynamic parameters of the ceramic sample of Ca1–2xBi2xMo1–xGexO4 were measured by the transmission line method. The average permittivity increases with x from ~10 to ~18 which correlates with theoretical ε, Vmol and total polarizability of samples. An increase in the concentration of bismuth and germanium leads to the additional resonant peaks in the spectra of ε, S11 and S21. These resonant peaks can be probably caused by the appearance of irregular structural domains of Bi atoms or induced by the increased size of grains in the ceramic samples.
Keywords
Full Text:
PDFReferences
Ramarao SD, Roopas Kiran S, Murthy VRK. Structural, lattice vibrational, optical and microwave dielectric studies on Ca1–xSrxMoO4 ceramics with scheelite structure. Mater Res Bull. 2014;56:71–79. doi:10.1016/j.materresbull.2014.04.064
Choi GK, Cho SY, An JS, Hong KS. Microwave dielectric properties and sintering behaviors of scheelite compound CaMoO4. J Eur Ceram Soc. 2006;26(10–11):2011–2015. doi:10.1016/j.jeurceramsoc.2005.09.051
Guo HH, Zhou D, Pang LX, Qi ZM. Microwave dielectric properties of low firing temperature stable scheelite structure (Ca,Bi)(Mo,V)O4 solid solution ceramics for LTCC applications. J Eur Ceram Soc. 2019;39(7):2365–2373. doi:10.1016/j.jeurceramsoc.2019.02.010
Choi GK, Kim JR, Yoon SH, Hong KS, Microwave dielectric properties of scheelite (A = Ca, Sr, Ba) and wolframite (A = Mg, Zn, Mn) AMoO4 compounds. J Eur Ceram Soc. 2007;27:(8–9):3063–3067. doi:10.1016/j.jeurceramsoc.2006.11.037
Huerta-Flores AM, Juarez-Ramirez I, Torres-Martinez LM, Carrera-Crespo JE, Gomez-Bustamante T, Sarabia-Ramos O. Synthesis of AMoO4 (A = Ca, Sr, Ba) photocatalysts and their potential application for hydrogen evolution and the degradation of tetracycline in water. J Photochem Photobiol A Chem. 2018;356:29–37. doi:10.1016/j.jphotochem.2017.12.029
Yao WF, Ye JH. Photophysical and photocatalytic properties of Ca1–xBixVxMo1–xO4 solid solutions. J Phys Chem B. 2006;110(23):11188–11195. doi:10.1021/jp0608729
Pang LX, Zhou D, Liu WG, Qi ZM, Yue ZX. Crystal structure and microwave dielectric behaviors of scheelite structured (1–x)BiVO4 – xLa2/3MoO4 (0.0 ≤ x ≤1.0) ceramics with ultra-low sintering temperature. J Eur Ceram Soc. 2018;38(5):1535–1540. doi:10.1016/j.jeurceramsoc.2017.10.051
Guo HH, Zhou D, Pang LX, Qi ZM. Microwave dielectric properties of low firing temperature stable scheelite structured (Ca,Bi)(Mo,V)O4 solid solution ceramics for LTCC applications. J Eur Ceram Soc. 2019;39(7):2365–2373. doi:10.1016/j.jeurceramsoc.2019.02.010
Mikhaylovskaya ZA, Buyanova ES., Petrova SA, Klimova AV. ABO4 type scheelite phases in (Ca/Sr)MoO4 – BiVO4 – Bi2Mo3O12 systems: synthesis, structure and optical properties. Chim Techno Acta. 2021;8(2):20218204. doi:10.15826/chimtech.2021.8.2.04
Zhang GG, Fang QF, Wang XP, Yi ZG. Dielectric relaxation study of Pb1–xLaxMoO4+δ (x = 0–0.3) oxide-ion conductors. J Phys Condens Matter. 2003;15(24):4135–4142. doi:10.1088/0953-8984/15/24/307
Zhou D, Li WB, Guo J, Pang LX, Qi ZM, Shao T, Xie HD, Yue ZX, Yao X. Structure, phase evolution, and microwave dielectric properties of (Ag0.5Bi0.5)(Mo0.5W0.5)O4 ceramic with ultralow sintering temperature. Inorg Chem. 2014;53(11):5712–5716. doi:10.1021/ic5004808
Shannon RD. Revised effective ionic radii and systematic studies of interatomic distances in halides and chalcogenides. Acta Cryst. 1976;A32:751–767. doi:10.1107/S0567739476001551
Ajiboye TO, Oyewo OA, Onwudiwe DC. The performance of bismuth-based compounds in photocatalytic applications. Surf Interfaces. 2021;23:100927. doi:10.1016/j.surfin.2021.100927
Ivanov MY, Nadolinny VA, Bagryanskaya EG, Grishin YA, Fedin MV, Veber SL. Bismuth germanate as a perspective material for dielectric resonators in EPR spectroscopy. J Magn Reson. 2016;271:83–89. doi:10.1016/j.jmr.2016.08.009
Mikhaylovskaya ZA, Abrahams I, Petrova SA, Buyanova ES, Tarakina NV, Morozova MV Structural, photocatalytic and electroconductive properties of bismuth-substituted CaMoO4. J Sol State Chem. 2020;291:121627. doi:10.1016/j.jssc.2020.121627
Kubelka P, Munk FZ Ein beitrag zur optik der farbanstriche. Techn Phys. 1931;12:593–601.
Salje EKH, Carpenter MA, Malcherek T, Boffa Ballaran T. Autocorrelation analysis of infrared spectra from minerals. Eur J Mineral. 2000;12(3):503–519. doi:10.1127/0935-1221/2000/0012-0503
Malkin A, Chechetkin V, Korotkov A, Knyazev NC. Estimation of uncertainty of permittivity measurement with transmission line method in the wide frequency range. In: The 29th Telecommunications Forum TELFOR; 2021 Nov 23–24; Belgrade, Serbia. p. 1–3. doi:10.1109/TELFOR52709.2021.9653335
Malkin AI, Knyazev NS, Dielectric permittivity and permeability measurement system. In: Radio Electronics & Information Technologies REIT; 2017 March 15; Yekaterinburg, Russia. p. 45–51.
Nicolson AM, Ross GF. Measurement of the intrinsic properties of materials by time-domain techniques. IEEE Trans Instrum Meas. 1970;19(4):377–82 doi:10.1109/TIM.1970.4313932
Sameera S, Prabhakar Rao P, Divya S, Raj KV, Aju Thara TR. High IR reflecting BiVO4–CaMoO4 based yellow pigments for cool roof applications. Energ Buildings. 2017;154:491–98. doi:10.1016/j.enbuild.2017.08.089
Porto SPS, Scott JF. Raman Spectra of CaWO4, SrWO4, CaMoO4, and SrMoO4. Phys Rev. 1967;157(3):716–719. doi:10.1103/physrev.157.716
Guo J, Randall CA, Zhang G, Zhou D, Chen Y, Wang H. Synthesis, structure, and characterization of new low-firing microwave dielectric ceramics: Ca1−3xBi2xФxMoO4. J Mater Chem C. 2014;2(35):7364–7372. doi:10.1039/c4tc00698d
Mikhaylovskaya ZA, Pankrushina EA, Komleva EV, Ushakov AV, Streltsov SV, Isaac Abrahams I, PetrovaSA. Effect of Bi substitution on the cationic vacancy ordering in SrMoO4-based complex oxides: structure and properties. Mater Sci Eng B. 2022;281:115741. doi:10.1016/j.mseb.2022.115741
Jayaraman A, Wang SY, Shieh SR, Sharma SK, Ming LC. High‐pressure Raman study of SrMoO4 up to 37 GPa and pressure‐induced phase transitions. J Raman Spectrosc. 1995;26(6):451–455. doi:10.1002/jrs.1250260609
Kanamori H., Hayashi S, Ikeda Y. External lattice vibration Modes in scheelites. J Phys Soc Jpn. 1974;36 (2):511–516. doi:10.1143/JPSJ.36.511
Verma A, Sharma SK. Rare-earth doped/codoped CaMoO4 phosphors: A candidate for solar spectrum conversion. Solid State Sci. 2019;96:105945. doi:10.1016/j.solidstatesciences.2019.105945
Shannon RD. Dielectric polarizabilities of ions in oxides and fluorides. J Appl Phys. 1993;73(1):348–66. doi:10.1063/1.353856
DOI: https://doi.org/10.15826/chimtech.2022.9.4.11
Copyright (c) 2022 Zoya A. Mikhaylovskaya, Elena S. Buyanova, Sofia A. Petrova, Elizaveta A. Pankrushina, Alexander I. Malkin, Alexey N. Korotkov, Nikolay S. Knyazev

This work is licensed under a Creative Commons Attribution 4.0 International License.
© Website Chimica Techno Acta, 2014–2024
ISSN 2411-1414 (Online)
This journal is licensed under a Creative Commons Attribution 4.0 International