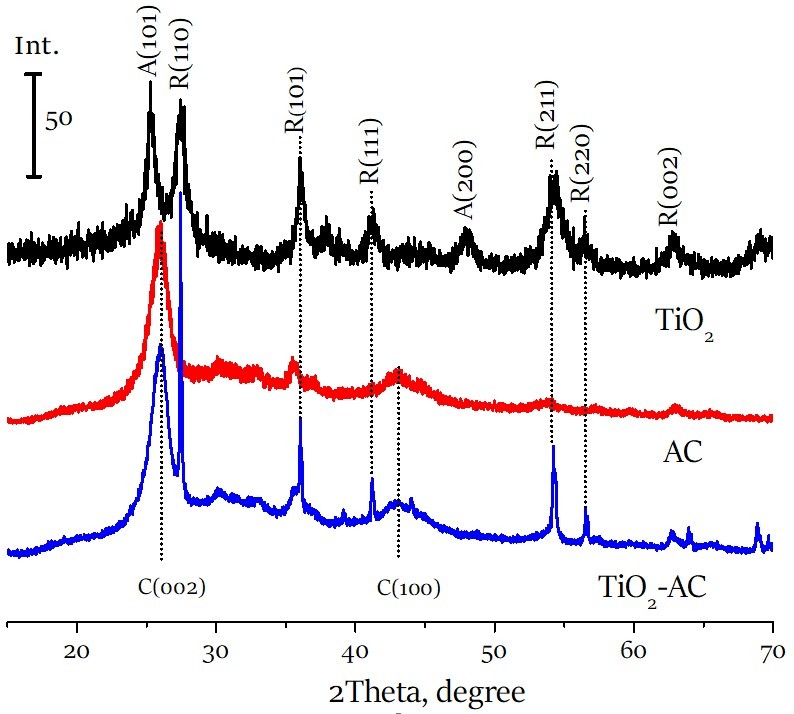
Titanium dioxide - activated carbon composite for photoelectrochemical degradation of phenol
Abstract
Keywords
Full Text:
PDFReferences
Albuquerque BR, Heleno SA, et al. Phenolic compounds: current industrial applications, limitations and future challenges. Food Funct. 2021;12(1):14–29. doi:10.1039/D0FO02324H
Xu N, Qiu C, et al. Analysis of phenol biodegradation in antibiotic and heavy metal resistant Acinetobacter lwoffii NL1. Front. Microbiol. 2021;12:725755. doi:10.3389/fmicb.2021.725755
Pardeshi SK, Patil AB. A simple route for photocatalytic degradation of phenol in aqueous zinc oxide suspension us-ing solar energy. Sol Energy. 2008;82(8):700–705. doi:10.1016/j.solener.2008.02.007
Gupta S, Ashrith G, et al. Acute phenol poisoning: A life-threatening hazard of chronic pain relief. Clin. Toxicol. 2008;46(3):250–253. doi:10.1080/15563650701438888
Kim HY, Kim OH, Sung MK. Effects of phenol-depleted and phenol-rich diets on blood markers of oxidative stress, and urinary excretion of quercetin and kaempferol in healthy volunteers. J Am Coll Nutr. 2003;22(3):217–223. doi:10.1080/07315724.2003.10719296
Villegas LGC, Mashhadi N, et al. A short review of tech-niques for phenol removal from wastewater. Curr Pollution Rep. 2016;2:157–167. doi:10.1007/s40726-016-0035-3
Li H, Yao Y, et al. Degradation of phenol by photocatalysis using TiO2/montmorillonite composites under UV light. Environ Sci Pollut Res. 2022. doi:10.1007/s11356-022-20638-8
Iervolino G, Zammit I, et al. Limitations and prospects for wastewater treatment by UV and visible-light-active heter-ogeneous photocatalysis: A critical review. Top Curr Chem (Z). 2020;378:7. doi:10.1007/s41061-019-0272-1
Li C, Wang WD. Photocatalytic degradation of phenol over MWCNTs-TiO2 composite catalysts with different diame-ters. Chin J Chem Phys. 2009;22(4):423–428. doi:10.1088/1674-0068/22/04/423-428
Velasco LF, Parra JB, Ania CO. Role of activated carbon fea-tures on the photocatalytic degradation of phenol. Appl Surf Sci. 2010;256(17):5254–5258. doi:10.1016/j.apsusc.2009.12.113
Malekshoar G, Pal K, et al. Enhanced solar photocatalytic degradation of phenol with coupled graphene-based titani-um dioxide and zinc oxide. Ind Eng Chem Res. 2014;53(49):18824–18832. doi:10.1021/ie501673v
Yao S, Li J, Shi Z. Immobilization of TiO2 nanoparticles on activated carbon fiber and its photodegradation perfor-mance for organic pollutants. Particuol. 2010;8(3):272–278. doi:10.1016/j.partic.2010.03.013
Nkwachukwu OV, Muzenda C, et al. Photoelectrochemical degradation of organic pollutants on a La3+ doped BiFeO3 perovskite. Catalysts. 2021;11(9):1069. doi:10.3390/catal11091069
Mukherjee A. Chakrabarty S, et al. Visible-light-mediated electrocatalytic activity in reduced graphene oxide-supported bismuth ferrite. ACS Omega. 2018;3(6):5946–5957. doi:10.1021/acsomega.8b00708
Bard AJ. Photoelectrochemistry. Sci. 1980;207(4427):139–144. doi:10.1126/science.207.4427.139
Barham JP, Köning B. Synthetic photoelectrochemistry. Angew Chem Int Ed. 2020;59:11732–11747.doi:10.1002/anie.201913767
Marwat MA, Humayun M, et al. Advanced catalysts for pho-toelectrochemical water splitting. ACS Appl Energy Mater. 2021;4(11):12007–12031. doi:10.1021/acsaem.1c02548
Villota-Zuleta JA, Rodríguez-Acosta, et al. Experimental data on the photoelectrochemical oxidation of phenol: Analysis of pH, potential and initial concentration. Data Brief. 2019;24:103949. doi:10.1016/j.dib.2019.103949
Liu S, Zhao X, et al. Peroxymonosulfate enhanced photoe-lectrocatalytic degradation of phenol activated by Co3O4 loaded carbon fiber cathode. J Catal. 2017;355:167–175. doi:10.1016/j.jcat.2017.09.016
Haro M, Velasco LF, Ania CO. Carbon-mediated photoin-duced reactions as a key factor in the photocatalytic per-formance of C/TiO2. Catal Sci Technol. 2012;2:2264–2272. doi:10.1039/C2CY20270K
Singh P, Singh R, et al. Effect of nanoscale TiO2-activated carbon composite on Solanum lycopersicum (L.) and Vigna radiata (L.) seeds germination. Energy Ecol Environ. 2016;1:131–140. doi:10.1007/s40974-016-0009-8
Xing B, Shi C, et al. Preparation of TiO2/activated carbon composites for photocatalytic degradation of RhB under UV light irradiation. J Nanomater. 2016;2016:8393648. doi:10.1155/2016/8393648
Haider A, Jameel ZN, Taha SY. Synthesis and characteriza-tion of TiO2 nanoparticles via sol-gel method by pulse laser ablation. In: The 5th International scientific Conference on Nanotechnology & Advanced Materials Their Applications; 2015 Nov 3–4; Baghdad, Iraq. p. 761–771.
Arabi-Katbi OI, Pratsins SE, et al. Monitoring the flame synthesis of TiO2 particles by in-situ FTIR spectroscopy and thermophoretic sampling. Combust Flame. 2001;124(4):560–572. doi:10.1016/S0010-2180(00)00227-3
Hanaor DAH, Sorrell CC. Review of the anatase to rutile phase transformation. J Mater Sci. 2011;46:855–874. doi:10.1007/s10853-010-5113-0
Kusiak-Nejman E, Wanag A, et al. Modification of titanium dioxide with graphitic carbon from anthracene thermal de-composition as a promising method for visible-active pho-tocatalysts preparation. J Adv Oxid Technol. 2016;19(2):227–235. doi:10.1515/jaots-2016-0206
Luttrell T, Halegamage S, et al. Why is anatase a better photocatalyst than rutile? – Model studies on epitaxial TiO2 films. Sci Rep. 2014;4:4043. doi:10.1038/srep04043
Liu Z, Jian Z, et al. Low-temperature reverse microemul-sion synthesis, characterization, and photocatalytic per-formance of nanocrystalline titanium dioxide. Int J Photo-energy. 2012;2012:702503. doi:10.1155/2012/702503
Zhang H, Wang X, et al. Synthesis and characterization of TiO2/graphene oxide nanocomposites for photoreduction of heavy metal ions in reverse osmosis concentrate. RSC Adv. 2018;8:34241–34251. doi:10.1039/C8RA06681G
Muhammad AS, Naser JT, et al. Photocatalytic degradation of methylene blue and phenol using TiO2/activated-carbon composite catalysts. Asian J Chem. 2015;27(1): 343–348. doi:10.14233/ajchem.2015.17936
Greco G, Mazzio KA, et al. Structural study of carbon-coated TiO2 anatase nanoparticles as high-performance anode ma-terials for Na-Ion batteries. ACS Appl Energy Mater. 2019;2(10):7142–7151. doi:10.1021/acsaem.9b01101
Wang H, Xia Y, et al. Highly active deficient ternary sulfide photoanode for photoelectrochemical water splitting. Nat Commun. 2020;11:3078. doi:10.1038/s41467-020-16800-w
Doménech-Carbó A, Doménech-Carbó MT, Costa V. Electro-chemical Methods in Archaeometry, Conservation and Res-toration. Springer-Verlag Berlin Heidelberg: New York; 2009. 199 p.
Jung H, Chae SY, et al. Effect of the Si/TiO2/BiVO4 hetero-junction on the onset potential of photocurrents for solar water oxidation. ACS Appl Mater Interfaces. 2015;7(10):5788–5796. doi:10.1021/am5086484
Bertolizzi L, Bisquert J. Equivalent circuit of electrons and holes in thin semiconductor films for photoelectrochemical water splitting applications. J Phys Chem Lett. 2012;3(17):2517–2522. doi:10.1021/jz3010909
Qu J, Li GR, Gao XP. One-dimensional hierarchical titania for fast reaction kinetics of photoanode materials of dye-sensitized solar cells. Energy Environ Sci. 2010;3:2003–2009. doi:10.1039/C003646C
Sun Y, Yan KP. Effect of anodization voltage on perfor-mance of TiO2 nanotube arrays for hydrogen generation in a two-compartment photoelectrochemical cell. Int J Hydrog Energy. 2014;22:11368–11375. doi:10.1016/j.ijhydene.2014.05.115
Grabowska E, Reszczyńska J, Zaleska. Mechanism of phenol photodegradation in the presence of pure and modified-TiO2: A review. Water Res. 2012;46(17):5453–5471. doi:10.1016/j.watres.2012.07.048
Mohamed A, Yousef S, et al. Rapid photocatalytic degrada-tion of phenol from water using composite nanofibers un-der UV. Environ Sci Eur. 2020;32:160.doi:10.1186/s12302-020-00436-0
Orudzhevz FF, Aliev ZM, et al. Photoelectrocatalytic oxida-tion of phenol on TiO2 nanotubes under oxygen pressure. Russ J Electrochem. 2015;51(12):1108–1114. doi:10.1134/S1023193515110130
Asenjo NG, Santamaría, et al. Correct use of the Langmuir–Hinshelwood equation for proving the absence of a synergy effect in the photocatalytic degradation of phenol on a sus-pended mixture of titania and activated carbon. Carbon. 2013;55:62–69. doi:10.1016/j.carbon.2012.12.010
Zamri MSFA, Sapwe N. Kinetic study on photocatalytic deg-radation of phenol using green electrosynthesized TiO2 na-noparticles. Materialstoday: Proceedings. 2019;19(4):1261–1266. doi:10.1016/j.matpr.2019.11.131
Alalm MG, Tawfik A, Ookawara S. Solar photocatalytic deg-radation of phenol by TiO2/AC prepared by temperature impregnation method. Desalination Water Treat. 2016;57(2):835–844. doi:10.1080/19443994.2014.969319
Prabha I, Lathasree S. Photodegradation of phenol by zinc oxide, titania and zinc oxide–titania composites: Nanopar-ticle synthesis, characterization and comparative photo-catalytic efficiencies. Mater Sci Semicond Process. 2014;26:603–613. doi:10.1016/j.mssp.2014.05.031
Thabet M, El-Zomrawy AA. Degradation of acid red 17 dye with ammonium persulphate in acidic solution using pho-toelectrocatalytic methods. Arab J Chem. 2016;9:S204–S208. doi:10.1016/j.arabjc.2011.03.001
Duan X, Ma F, et al. Electrochemical degradation of phenol in aqueous solution using PbO2 anode. J Taiwan Inst Chem Eng. 2013;44:95–102. doi:10.1016/j.jtice.2012.08.009
Neumann-Spallart M, Shinde SS, et al. Photoelectrochemi-cal degradation of selected aromatic molecules. Electro-chim Acta. 2013;111:830–836. doi:10.1016/j.electacta.2013.08.080
Katada M, Fujii A. Infrared spectroscopy of protonated phe-nol–water clusters. J Phys Chem A. 2018;122(27):5822–5831. doi:10.1021/acs.jpca.8b04446
Granucci G, Hynes JT, et al. A theoretical investigation of excited-state acidity of phenol and cyanophenols. J Am Chem Soc. 2000;122(49):12243–12253. doi:10.1021/ja993730j
Balaska A, Samar ME, Grid A. Phenol photodegradation process assisted with Wells–Dawson heteropolyacids. De-salination Water Treat. 2015;54(2):382–392. doi:10.1080/19443994.2014.883577
Dubale AA, Ahmed IN, et al. A highly stable metal–organic framework derived phosphorus doped carbon/Cu2O struc-ture for efficient photocatalytic phenol degradation and hydrogen production. J Mater Chem A. 2019;7:6062–6079. doi:10.1039/C8TA12544A
DOI: https://doi.org/10.15826/chimtech.2022.9.4.16
Copyright (c) 2022 L. H. Q. Anh, Uyen P. N. Tran, P. V. G. Nghi, H. T. Le, N. T. B. Khuyen, T. D. Hai

This work is licensed under a Creative Commons Attribution 4.0 International License.
© Website Chimica Techno Acta, 2014–2024
ISSN 2411-1414 (Online)
This journal is licensed under a Creative Commons Attribution 4.0 International