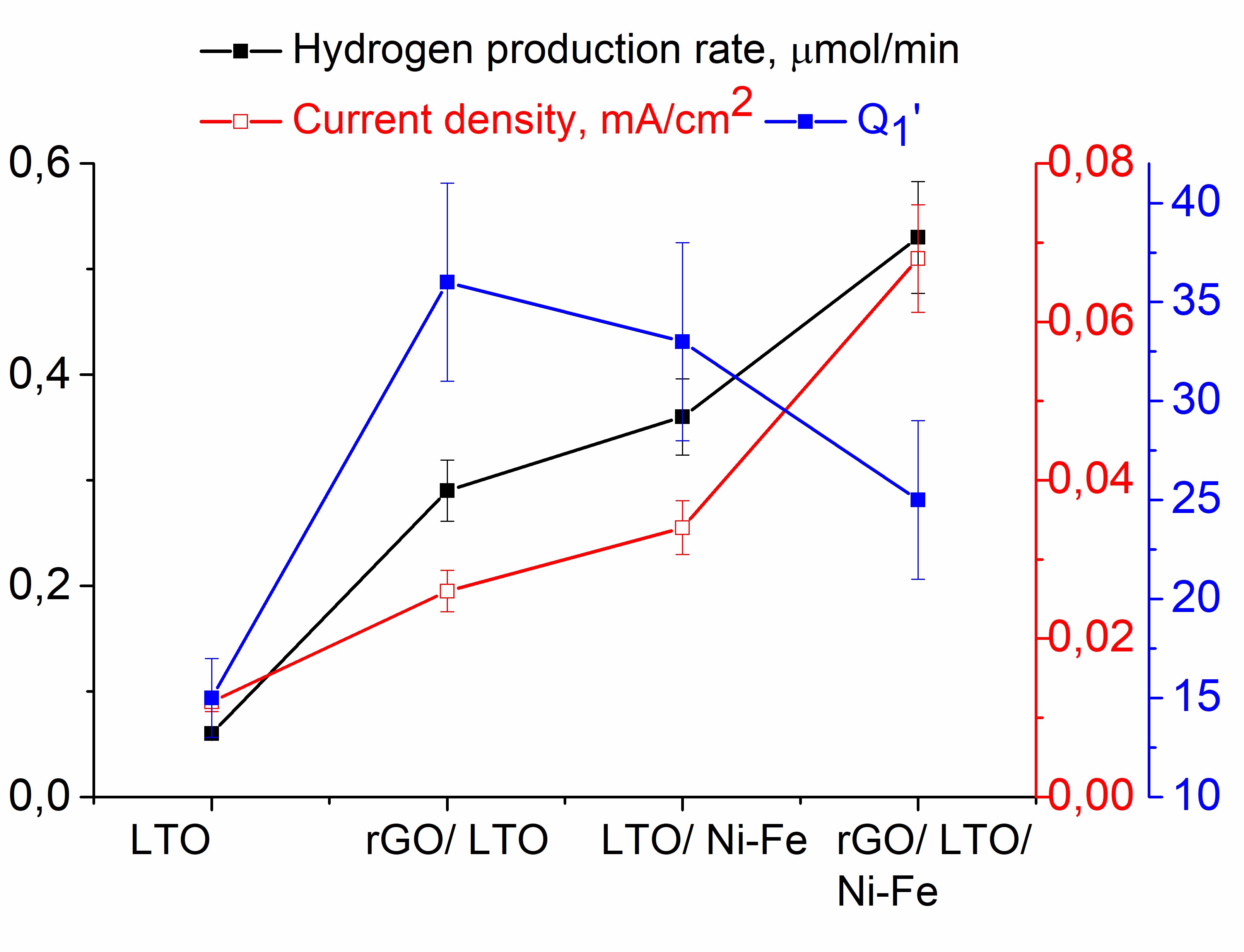
Application of the similarity theory to analysis of photocatalytic hydrogen production and photocurrent generation
Abstract
Keywords
Full Text:
PDFReferences
Turner JA. Sustainable hydrogen production. Sci. 2004;305(5686):972–974. doi:10.1126/science.1103197
Fujishima A, Honda K. Electrochemical рhotolysis of water at a semiconductor electrode. Nat. 1972;238:37–38. doi:10.1038/238037a0
Vorontsov AV, Kozlova EA, Besov AS, Kozlov DV, Kiselev SA, Safatov AS. Photocatalysis: light energy conversion for the oxidation, disinfection, and decomposition of water. Kin Cat. 2010;51(6):801–808. doi:10.1134/S0023158410060042
Tomboc GM, Gadisa BT, Joo J, Kim H, Lee K. Hollow structured metal sulfides for photocatalytic hydrogen generation. ChemNanoChem. 2020;6(6):850–869. doi:10.1002/cnma.202000125
Stolarczyk JK, Bhattacharyya S, Polavarapu L, Feldmann J. Challenges and prospects in solar water splitting and CO2 reduction with inorganic and hybrid nanostructures. ACS Catal. 2018;8(4):3602–3635. doi:10.1021/acscatal.8b00791
Li X, Wang W, Dong F, Zhang Z, Han L, Luo X, Huang J, Feng Z, Chen Z, Jia G, Zhang T. Recent advances in noncontact external-field-assisted photocatalysis: from fundamentals to applications. ACS Catal. 2021;11(8):4739–4769. doi:10.1021/acscatal.0c05354
Krjukov AI, Strojuk AL, Kuchmij SYa, Pohodenko VD. Nanofotokataliz [Nanophotocatalysis]. Kiev: Akademperiodika; 2013. 618 p. Russian.
Cao S, Wang C-J, Fu W-F, Chen Y. Metal phosphides as co-catalysts for photocatalytic and photoelectrocatalytic water splitting. ChemSusChem. 2017;10(22):4306–4323. doi:10.1002/cssc.201701450
Carp O, Huisman CL, Reller A. Photoinduced reactivity of titanium dioxide. Prog Solid State Ch. 2004;32:33–177. doi:10.1016/j.progsolidstchem.2004.08.001
Frank-Kamenetsky DA. Osnovy makrokinetiki. Diffuziya i teploperedacha v himicheskoj kinetike [Fundamentals of macrokinetics. Diffusion and heat transfer in chemical kinetics]. Dolgoprudny: Izdatel’skij dom “Intellekt”; 2008. 408 p. Russian.
Sedov LI. Metody podobiya i razmernosti v mehanike [Similarity and dimensionality methods in mechanics]. Moscow: Nauka; 1977. 440 p. Russian.
Liu Z, Yu Y, Zhu X, Fang J, Xu W, Hu X, Li R, Yao L, Qin J, Fang Z. Semiconductor heterojunctions for photocatalytic hydrogen production and Cr(VI) reduction: a review. Mater Res Bull. 2022;147:111636. doi:10.1016/j.materresbull.2021.111636
Ismael M. Latest progress on the key operating parameters affecting the photocatalytic activity of TiO2-based photocatalysts for hydrogen fuel production: a comprehensive review. Fuel. 2021;303:121207. doi:10.1016/j.fuel.2021.121207
Vyas Y, Chundawat P, Dharmendra D, Punjabi PB, Ameta C. Review on hydrogen production photocatalytically using carbon quantum dots: future fuel. Int J Hydrog Energy. 2021;46(75):37208–37241. doi:10.1016/j.ijhydene.2021.09.004
Kozlova EA, Lyulyukin MN, Markovskaya DV, Selishchev DS, Cherepanova SV, Kozlov DV. Synthesis of Cd1−xZnxS photocatalysts for gas-phase CO2 reduction under visible light. Photoch Photobio Sci. 2019;18(4):871–877. doi:10.1039/c8pp00332g
Tasleem S, Tahir M. Recent progress in structural development and band engineering of perovskites materials for photocatalytic solar hydrogen production: a review. Int J Hydrog Energy. 2020;45(38):19078–19111. doi:10.1016/j.ijhydene.2020.05.090
Al-Ahmed A. Photocatalytic properties of graphitic carbon nitrides (g-C3N4) for sustainable green hydrogen production: recent advancement. Fuel. 2022;316:123381. doi:10.1016/j.fuel.2022.123381
Singla S, Sharma S, Basu S, Shetti NP, Aminabhavi TM. Photocatalytic water splitting hydrogen production via environmental benign carbon based nanomaterials. Int. J. Hydrogen Energy. 2021;46(68):33696–33717. doi:10.1016/j.ijhydene.2021.07.187
Lv Y, Chen P, Foo JJ, Zhang J, Qian W, Chen C, Ong W-J. Dimensionality-dependent MoS2 toward efficient photocatalytic hydrogen evolution: from synthesis to modifications in doping, surface and heterojunction engineering. Mater Today Nano. 2022;18:10019. doi:10.1016/j.mtnano.2022.100191
Kozlova EA, Parmon VN. Heterogeneous semiconductor photocatalysts for hydrogen production from aqueous solutions of electron donors. Russ Chem Rev. 2017;86(9):870–906. doi:10.1070/RCR4739
Braslavsky SE, Braun AM, Cassano AE, Emeline AV, Litter MI, Palmisano L, Parmon VN, Serpone N. Glossary of terms used in photocatalysis and radiation catalysis (IUPAC Recommendations 2011). Pure Appl Chem. 2011;83(4):931–1014. doi:10.1351/PAC-REC-09-09-36
Lakhera SK, Rajan A, Rugma TP, Bernaurdshaw N. A review on particulate photocatalytic hydrogen production system: Progress made in achieving high energy conversion efficiency and key challenges ahead. Renew Sustain Ener Rev. 2021;152:11169. doi:10.1016/j.rser.2021.111694
Boreskov GK. Geterogennyj kataliz [Heterogeneous catalysis]. Moscow: Nauka; 1986. 304 p. Russian.
Nakamura A, Ota Y, Koike K, Hidaka Y, Nishioka K, Sugiyama M, Fujii K. A 24.4% solar to hydrogen energy conversion efficiency by combining concentrator photovoltaic modules and electrochemical cells. Appl Phys Expess. 2015;8:107101. doi:10.7567/apex.8.107101
Ziegenbalg D, Pannwitz A, Rau S, Dietzek-Ivanšić B, Streb C. Comparative evaluation of light-driven catalysis: a framework for standardized reporting of data. Angew Chem-Ger Edit. 2022;61(28):e202114106. doi:10.1002/anie.202114106
Chen S, Liu T, Zheng Z, Ishaq M, Liang G, Fan P, Chen T, Tang J. Recent progress and perspectives on Sb2Se3-based photocathodes for solar hydrogen production via photoelectrochemical water splitting. J Energy Chem. 2022;67:508–523. doi:10.1016/j.jechem.2021.08.062
Lewerenz HJ. Micro- and nanotopographies for photoelectrochemical energy conversion. I: The photovoltaic mode. Electrochim Acta. 2011;56(28):10713–10725. doi:10.1016/j.electacta.2011.05.026
Ahmed M, Dincer I. A review on photoelectrochemical hydrogen production systems: Challenges and future directions. Int J Hydrog Energy. 2019;44(5):2474–2507. doi:10.1016/j.ijhydene.2018.12.037
Esmaili H, Kowsari E, Tafreshi SS, Ramakrishna S, de Leeuw NH, Abdouss M. TiO2 nanoarrays modification by a novel Cobalt-heteroatom doped graphene complex for photoelectrochemical water splitting: An experimental and theoretical study. J Mol Liq. 2022;356:118960. doi:10.1016/j.molliq.2022.118960
Chavan GT, Shinde NM, Sabah FA, Patil SS, Sikora A, Prakshale VM, Kamble SS, Chaure NB, Deshmukh LP, Kim A, Jeon C-W. Chemical synthesis of Cd1–x–yZnxCuySzSe1–z composite thin films for photoelectrochemical solar cell. Appl Surf Sci. 2022;574:2022. doi:10.1016/j.apsusc.2021.151581
Chatterjee P, Ambati MSK, Chakraborty AK, Chakrabortty S, Biring S, Ramakrishna S, Wong TKS, Kumar A, Lawaniya R, Dalapati GK. Photovoltaic/photo-electrocatalysis integration for green hydrogen: A review. Energ Convers Manage. 2022;261:115648. doi:10.1016/j.enconman.2022.115648
Ikram A, Zulfequar M, Satsangi VR. Role and prospects of green quantum dots in photoelectrochemical hydrogen generation: A review. Int J Hydrog Energy. 2022;47(22):11472–11491. doi:10.1016/j.ijhydene.2022.01.187
Lee SY, Oh J-Y, Patil RP, Kim M, Jang JS, Jin H, Kim S, Lee HJ. A general guide for adsorption of cadmium sulfide (CdS) quantum dots by successive ionic layer adsorption and reaction (SILAR) for efficient CdS-sensitized photoelectrochemical cells. Appl Surf Sci. 2022;589:152898. doi:10.1016/j.apsusc.2022.152898
Hogerwaard J, Dincer I, Naterer GF. Experimental investigation and optimization of integrated photovoltaic and photoelectrochemical hydrogen generation. Energ Convers Manage. 2020;207:112541. doi:10.1016/j.enconman.2020.112541
Shiyani T, Mahapatra SK, Banerjee I. Basil sensitized ZnO photoelectrochemical cell for solar energy conversion. Mater Today-Proc. 2020;32(3):412–416. doi:10.1016/j.matpr.2020.02.089
Shang J, Gao Y, Chen H, Wang X. Top-down fabrication of bromine doped Bi2O3 nanowires with efficient charge separation for photoelectrochemical application. Physica B: Condensed Matter. 2020;599:412587. doi:10.1016/j.physb.2020.412587
Ali M, Pervaiz E, Sikandar U, Khan Y. A review on the recent developments in zirconium and carbon-based catalysts for photoelectrochemical water-splitting. Int J Hydrog Energy. 2021;46(35):18257–18283. doi:10.1016/j.ijhydene.2021.02.202
Knapik A, Syrek K, Kozieł M, Zaraska L. Cathodic deposition of SnO2 layers on transparent conductive substrates and their photoelectrochemical activity. J Ind Eng Chem. 2022;111:380–388. doi:10.1016/j.jiec.2022.04.020
Zhang Y, Bu Y, Wang L, Ao J-P. Regulation of the photogenerated carrier transfer process during photoelectrochemical water splitting: A review. Green Energy Environ. 2021;6(4):479–495. doi:10.1016/j.gee.2020.11.007
Hashim ET, Abdulameer A. Temperature effect on power drop of different photovoltaic modules. J Eng. 2016;22(5):129–143. doi:10.31026/j.eng.2016.05.09
Gurevich YuYa, Pleskov YuV. Photoelectrohimiya poluprovodnikov [Photoelectrochemistry of semiconductors]. Moscow: Nauka; 1983. 312 p. Russian.
Kamat PV, Tvrdy K, Baker DR, Padich JG. Beyong photovoltaics: semiconductor nanoarchitectures for liquid-junction solar cells. Chem Rev. 2010;110:6664–6688. doi:10.1021/cr100243p
Chen Z, Jaramillo TF, Deutsch TG, Kleiman-Shwarsctein A, Forman AJ, Gaillard N, Garland R, Takanabe K, Heske C, Sunkara M, McFarland EW, Domen K, Miller EL, Turner JA, Dinh HN. Accelerating materials development for photoelectrochemical hydrogen production: Standards for methods, definitions, and reporting protocols. J Mater Res. 2010;25:3–16. doi:10.1557/JMR.2010.0020
Strenk F. Peremeshivanije i apparaty s meshalkami [Mixing and devices with agitators]. Leningrad: Himiya; 1975. 384 p. Russian.
Antoniadou M, Daskalaki VM, Balis N, Kondarides DI, Kordulis C, Lianos P. Photocatalysis and photoelectrocatalysis using (CdS-ZnS)/TiO2 combined photocatalysts. Appl Catal B-Environm. 2011;107(1–2):188–196. doi:10.1016/j.apcatb.2011.07.013
Markovskaya DV, Zhurenok AV, Cherepanova SV, Kozlova EA. Solid solutions of CdS and ZnS: comparing photocatalytic activity and photocurrent generation. Appl Surf Sci Adv. 2021;4:100076. doi:10.1016/j.apsadv.2021.100076
Du S, Lin X, Li C, Li G, Zheng B, Liu Y, Xu H, Fang P. CoSe2 modified Se-decorated CdS nanowire Schottky heterojunctions for highly efficient photocatalytic hydrogen evolution. Chem Eng J. 2020;389:124431. doi:10.1016/j.cej.2020.124431
Li H, Wang Z, He Y, Meng S, Xu Y, Chen S, Fu X. Rational synthesis of MnxCd1–xS for enhanced photocatalytic H2 evolution: Effects of S precursors and the feed ratio of Mn/Cd on its structure and performance. J Colloid Interf Sci. 2019;535:469–480. doi:10.1016/j.jcis.2018.10.018
Zhao Y, Yang P, Li J. Fabrication of one-dimensional MnxCd1–xS@D–MoSeyS2–y heterostructure with enhanced visible-light photocatalytic hydrogen evolution. Int J Hydrog Energy. 2021;46(43):22422–22433. doi:10.1016/j.ijhydene.2021.04.063
Guo P, Zhang D, Liu X, Liu W, Wang R, Zhang Z, Qiu S. In situ self-assembly of mesoporous Zn-Cd-Mo-S quaternary metal sulfides with double heterojunction synergistic charge transfer for boosting photocatalytic hydrogen production. J Alloy Compd. 2022;921:166066. doi:10.1016/j.jallcom.2022.166066
Kalia R, Pushpendra, Kunchala RK, Achary SN, Naidu BS. New insights on photocatalytic hydrogen evolution of ZnFe2−xGaxO4 (0 ≤x ≤ 2) solid solutions: Role of oxygen vacancy and ZnO segregated phase. J Alloy Compd. 2021;875:159905. doi:10.1016/j.jallcom.2021.159905
Ren B, Luan Q, Ma LL, Ding Y, Ma D, Cao X, Guo Y, Guan R, Chen Q. Amorphous domain induced LSPR Zn–Cr–In–S solid solution with enhanced visible photocatalytic H2 production. Mater Chem Phys. 2022;285:126100. doi:10.1016/j.matchemphys.2022.126100
Zhao C, Jiang H, Liang Q, Zhou M, Zhang Y, Li Z, Xu S. NH2-UiO-66 with heterogeneous pores assists zinc indium sulfide in accelerating the photocatalytic H2 evolution under visible-light irradiation. Sol Energy. 2020;207:599–608. doi:10.1016/j.solener.2020.07.005
Liu X, Wen D, Liu Z, Wei J, Bu D, Huang S. Thiocyanate-capped CdSe@Zn1–XCdXS gradient alloyed quantum dots for efficient photocatalytic hydrogen evolution. Chem Eng J. 2020;402:126178. doi:10.1016/j.cej.2020.126178
Huang J, Tao J, Liu G, Lu L, Tang H, Qiao G. In situ construction of 1D CdS/2D Nb2CTx MXene Schottky heterojunction for enhanced photocatalytic hydrogen production activity. Appl Surf Sci. 2022;573:151491. doi:10.1016/j.apsusc.2021.151491
Sk S, Tiwari A, Abraham BM, Manwar N, Perupogu V, Pal U. Constructing Cu/BN@PANI ternary heterostructure for efficient photocatalytic hydrogen generation: A combined experimental and DFT studies. Int J Hydrog Energy. 2021;46(54):27394–27408. doi:10.1016/j.ijhydene.2021.06.033
Wang Y, Guo S, Xin X, Zhang Y, Wang B, Tang S, Li X. Effective interface contact on the hierarchical 1D/2D CoO/NiCo-LDH heterojunction for boosting photocatalytic hydrogen evolution. Appl Surf Sci. 2021;549:149108. doi:10.1016/j.apsusc.2021.149108
Kim Y, Coy E, Kim H, Mrówczyński R, Torruella P, Jeong D-W, Choi KS, Jang JH, Song MY, Jang D-J, Peiro F, Jurga S, Kim HJ. Efficient photocatalytic production of hydrogen by exploiting the polydopamine-semiconductor interface. Appl Catal B-Environ. 2021;280:119423. doi:10.1016/j.apcatb.2020.119423
Xu G, Lin X, Tong Y, Du H, Gu L, Yuan Y. UiO-66 MOFs as electron transport channel to short circuit dye photosensitizer and NiS2 co-catalyst for increased hydrogen generation. Mater Lett. 2019;255:126593. doi:10.1016/j.matlet.2019.126593
Wang C, Fan H, Ren X, Wen Y, Wang W. Highly dispersed PtO nanodots as efficient co-catalyst for photocatalytic hydrogen evolution. Appl Surf Sci. 2018;462:423–431. doi:10.1016/j.apsusc.2018.08.126
Gogoi D, Koyani R, Golder AK, Peela NR. Enhanced photocatalytic hydrogen evolution using green carbon quantum dots modified 1-D CdS nanowires under visible light irradiation. Sol Energy. 2020;208:966–977. doi:10.1016/j.solener.2020.08.061
Yang C, Zhu Y, Liu Y, Wang H, Yang D. Ternary red phosphorus/CoP2/SiO2 microsphere boosts visible-light-driven photocatalytic hydrogen evolution from pure water splitting. J Mater Sci Technol. 2022;125:59–66. doi:10.1016/j.jmst.2022.02.034
Liu H, Chen J, Guo W, Xu Q, Min Y. A high efficiency water hydrogen production method based on CdS/WN composite photocatalytic. J Colloid Interf Sci. 2022;613:652–660. doi:10.1016/j.jcis.2022.01.014
Xu Z, Guo C, Liu X, Li L, Wang L, Xu H, Zhang D, Li C, Li Q, Wang W. Ag nanoparticles anchored organic/inorganic Z-scheme 3DOMM-TiO2–x-based heterojunction for efficient photocatalytic and photoelectrochemical water splitting. Chin J Catal. 2022;43(5):1360–1370. doi:10.1016/S1872-2067(21)63978-5
Tian Z, Yang X, Chen Y, Huang H, Hu J, Wen B. Fabrication of alveolate g-C3N4 with nitrogen vacancies via cobalt introduction for efficient photocatalytic hydrogen evolution. Int J Hydrog Energy. 2020;45(46):24792–24806. doi:10.1016/j.ijhydene.2020.06.274
Ma X, Li W, Li H, Dong M, Li X, Geng L, Fan H, Li Y, Qiu H, Wang T. Fabrication of novel and noble-metal-free MoP/In2S3 Schottky heterojunction photocatalyst with efficient charge separation for enhanced photocatalytic H2 evolution under visible light. J Colloid Interf Sci. 2022;617:284–292. doi:10.1016/j.jcis.2022.03.021
Liang Z, Yang C, Lu J, Dong X. Ultrathin Fe2P nanosheet co-catalyst CdS nanorod: The promising photocatalyst with ultrahigh photocatalytic H2 production activity. Appl Surf Sci. 2021;566:150732. doi:10.1016/j.apsusc.2021.150732
Zhong Y, Yang S, Zhang S, Cai X, Gao Q, Yu X, Xu Y, Zhou X, Peng F, Fang Y. CdS branched TiO2: Rods-on-rods nanoarrays for efficient photoelectrochemical (PEC) and self-bias photocatalytic (PC) hydrogen production. J Power Sources. 2019;430:32–42. doi:10.1016/j.jpowsour.2019.04.116
Boppella R, Choi CH, Moon J, Kim DH. Spatial charge separation on strongly coupled 2D-hybrid of rGO/La2Ti2O7/NiFe-LDH heterostructures for highly efficient noble metal free photocatalytic hydrogen generation. Appl Catal B-Environ. 2018;239:178–186. doi:10.1016/j.apcatb.2018.07.063
Guo C, Tian K, Wang L, Liang F, Wang F, Chen D, Ning J, Zhong Y, Hu Y. Approach of fermi level and electron-trap level in cadmium sulfide nanorods via molybdenum doping with enhanced carrier separation for boosted photocatalytic hydrogen production. J Colloid Interf Sci. 2021;583:661–671. doi:10.1016/j.jcis.2020.09.093
Rabell GO, Cruz MRA, Juárez-Ramírez I.Photoelectrochemical (PEC) analysis of ZnO/Al photoelectrodes and its photocatalytic activity for hydrogen production. Int J Hydrog Energy. 2022;47(12): 7770–7782. doi:10.1016/j.ijhydene.2021.12.107
Huang K, Li C, Zhang X, Wang L, Wang W, Meng X. Self-assembly synthesis of phosphorus-doped tubular g-C3N4/Ti3C2 MXene Schottky junction for boosting photocatalytic hydrogen evolution. Green Energy Environ. 2023;8(1):233–245. doi:10.1016/j.gee.2021.03.011
Mahvelati-Shamsabadi T, Fattahimoghaddam H, Lee B-K, Ryu H, Jang JI. Caesium sites coordinated in Boron-doped porous and wrinkled graphitic carbon nitride nanosheets for efficient charge carrier separation and Transfer: Photocatalytic H2 and H2O2 production. Chem Eng J. 2021;423:130067. doi:10.1016/j.cej.2021.130067
Deng Q, Ba G, Huo T, Li H, Hou W. Atomic carbon chain-linked polymeric carbon nitride: Roles of the carbon chain in enhancing the photocatalytic hydrogen evolution performance. Appl Catal A-Gen. 2020;606:117833. doi:10.1016/j.apcata.2020.117833
Zhao H, Fu H, Yang X, Xiong S, Han D, An X. MoS2/CdS rod-like nanocomposites as high-performance visible light photocatalyst for water splitting photocatalytic hydrogen production. Int J Hydrog Energy. 2022;47(13):8247–8260. doi:10.1016/j.ijhydene.2021.12.171
Li X, Hu J, Yang T, Yang X, Qu J, Li CM. Efficient photocatalytic H2-evolution coupled with valuable furfural-production on exquisite 2D/2D LaVO4/g-C3N4 heterostructure. Nano Energy. 2022;92:106714. doi:10.1016/j.nanoen.2021.106714
Sun B, Qiu P, Liang Z, Xue Y, Zhang X, Yang L, Cui H, Tian J. The fabrication of 1D/2D CdS nanorod@Ti3C2 MXene composites for good photocatalytic activity of hydrogen generation and ammonia synthesis. Chem Eng J. 2021;406:127177 doi:10.1016/j.cej.2020.127177
Zhang Q, Chen D, Song Q, Zhou C, Li D, Tian D, Jiang D. Holey defected TiO2 nanosheets with oxygen vacancies for efficient photocatalytic hydrogen production from water splitting. Brit Cer Pr. 2021;23:100979. doi:10.1016/j.surfin.2021.100979
Zhang K, Mou Z, Cao S, Wu S, Xu X, Li C. Well-designed NiS/CdS nanoparticles heterojunction for efficient visible-light photocatalytic H2 evolution. Int J Hydrog Energy. 2022;47(25):12605–12614. doi:10.1016/j.ijhydene.2022.02.009
Attia S, Helaïli N, Bessekhouad Y, Trari M. Physical properties of the solid solution CuFe2–xMnxO4 prepared by sol-gel route: Application to photocatalytic hydrogen production. J Photoch Photobio A. 2022;426:113745. doi:10.1016/j.jphotochem.2021.113745
Markovskaya DV, Zhurenok AV, Kozlova EA. Rate of photocatalytic hydrogen evolution and photovoltaic characteristics as a function of the nature and concentration of the electrolyte. Rus J Phys Chem A. 2022;96(5):1093–1098. doi:10.1134/S003602442205020X
DOI: https://doi.org/10.15826/chimtech.2023.10.2.03
Copyright (c) 2023 Dina V. Markovskaya, Ekaterina A. Kozlova

This work is licensed under a Creative Commons Attribution 4.0 International License.
Chimica Techno Acta, 2014–2025
eISSN 2411-1414
Copyright Notice