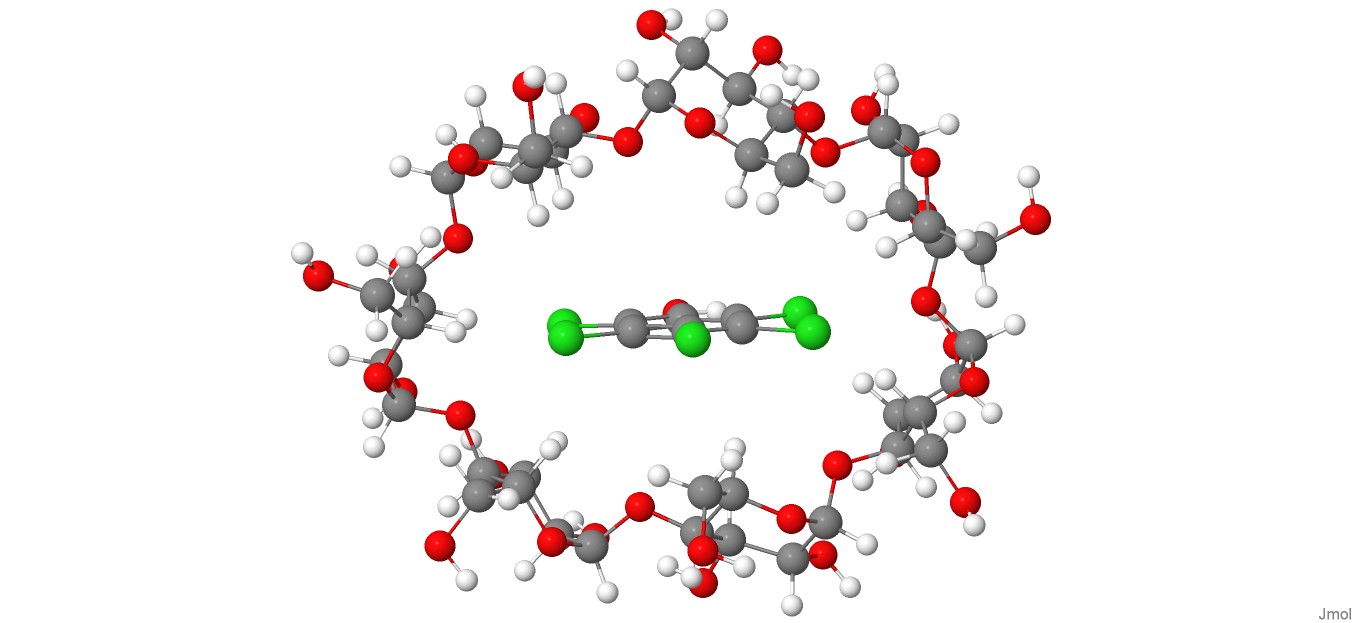
A DFT-D4 investigation of the complexation phenomenon between pentachlorophenol and β-cyclodextrin
Abstract
Keywords
Full Text:
PDFReferences
Mattia E, Otto S. Supramolecular systems chemistry. Nat Nanotechnol. 2015;10:111–119. doi:10.1038/nnano.2014.337
Kolesnichenko IV, Anslyn EV. Practical applications of supramolecular chemistry. Chem Soc Rev. 2017;46:2385–2390. doi:10.1039/C7CS00078B
Ma X, Zhao Y. Biomedical applications of supramolecular systems based on host–guest interactions. Chem Rev. 2014;115:7794–7839. doi:10.1021/cr500392w
Sambrook MR, Notman S. Supramolecular chemistry and chemical warfare agents: From fundamentals of recognition to catalysis and sensing. Chem Soc Rev. 2013;42:9251–9267. doi:10.1039/C3CS60230C
Bohmer V. Calixarenes, Macrocycles with (Almost) Unlimited Possibilities. Angew Chem Int Ed Engl. 1995;34:713–725. doi:10.1002/anie.199507131
Chen JF, Ding JD, Wei TB. Pillararenes: Fascinating planar chiral macrocyclic arenes. Chem Commun. 2021;57:9029–9039. doi:10.1039/D1CC03778A
Gerasko OA, Samsonenko DG, Fedin VP. Supramolecular chemistry of cucurbiturils. Russ Chem Rev. 2002;71:741–760. doi:10.1070/RC2002v071n09ABEH000748
Crini G. Review: a history of cyclodextrins. Chem Rev. 2014;114:10940–10975. doi:10.1021/cr500081p
Del Valle EM. Cyclodextrins and their uses: a review. Process Biochem. 2004;39(9):1033-1046. doi:10.1016/S0032-9592(03)00258-9
Crini G, Fourmentin S, Fenyvesi É, Torri G, Fourmentin M, Morin-Crini N. Cyclodextrins from molecules to applications. Environ Chem Lett. 2018;16:1361–1375. doi:10.1007/s10311-018-0763-2
Gidwani B, Vyas A. Pharmacokinetic study of solid-lipid-nanoparticles of altretamine complexed epichlorohydrin-β-cyclodextrin for enhanced solubility and oral bioavailability. Int J Biol Macromol. 2017;101:24–31. doi:10.1016/j.ijbiomac.2017.03.047
Alizadeh N, Malakzadeh S. Changes in chemical stability and bioactivities of curcumin by forming inclusion complexes of beta- and Gama-cyclodextrins. J Polym Res. 2020;27:42. doi:10.1007/s10965-019-1994-z
Yadav M, Thakore S, Jadeja R. A review on remediation technologies using functionalized Cyclodextrin. Environ Sci Pollut Res. 2022;29:236–250. doi:10.1007/s11356-021-15887-y
Roy I, Stoddart JF. Cyclodextrin metal–organic frameworks and their applications. Acc Chem Res. 2021;54(6):1440–1453. doi:10.1021/acs.accounts.0c00695
Liu Y, Lin T, Cheng C, Wang Q, Lin S, Liu C, Han X. Research progress on synthesis and application of cyclodextrin polymers. Molec. 2021;26(4):1090. doi:10.3390/molecules26041090
Bautista‐Renedo JM, Hernández‐Esparza R, Cuevas‐Yañez E, Reyes‐Pérez H, Vargas R, Garza J, González‐Rivas N. Deformations of cyclodextrins and their influence to form inclusion compounds. Int J Quantum Chem. 2021;122(6):e26859. doi:10.1002/qua.26859
Tian B, Hua S, Tian Y, Liu J. Cyclodextrin-based adsorbents for the removal of pollutants from wastewater: a review. Environ Sci Pollut Res. 2021;28:1317–1340. doi:10.1007/s11356-020-11168-2
Majd M, Yazdanpanah M, Bayatloo MR, Nojavan S. Recent advances and applications of cyclodextrins in magnetic solid phase extraction. Talanta. 2021;229:122296. doi:10.1016/j.talanta.2021.122296
Nelumdeniya NRM, Ranatunga RJKU. Complex forming behaviour of α, β and γ-cyclodextrins with varying size probe particles in silico. Ceylon J Sci. 2021;50(5):329–339. doi:10.4038/cjs.v50i5.7922
United States Environmental Protection Agency. Pentachlorophenol. Available from: https://www.epa.gov/ingredients-used-pesticide-products/pentachlorophenol
United States Environmental Protection Agency. Priority Pollutant List, 2014. Available from: https://www.epa.gov/sites/default/files/2015-09/documents/priority-pollutant-list-epa.pdf
Agency for Toxic Substances and Disease Registry (ATSDR), 2001. Toxicological Profile for Pentachlorophenol Atlanta, GA: U.S. Available from: https://semspub.epa.gov/work/10/100006534.pdf
Kraševec I, Nemeček N, Lozar Štamcar M, Kralj Cigić I, Prosen H. Non-destructive detection of pentachlorophenol residues in historical wooden objects. Polymers. 2021;13(7):1052. doi:10.3390/polym13071052
Jambhekar SS, Breen P. Cyclodextrins in pharmaceutical formulations I: structure and physicochemical properties, formation of complexes, and types of complex. Drug Discov Today. 2016;21:356–362. doi:10.1016/j.drudis.2015.11.017
Iacovino R, Rapuano F, Caso JV, Russo A, Lavorgna M, Russo C, Isidori M, Russo L, Malgieri G, Isernia C. β-Cyclodextrin inclusion complex to improve physicochemical properties of pipemidic acid: Characterization and bioactivity evaluation. Int J Mol Sci. 2013;14:13022–13041. doi:10.3390/ijms140713022
Bakó I, Jicsinszky L. Semiempirical calculations on cyclodextrins. J Incl Phenom Macrocycl Chem. 1994;18:275–289. doi:10.1007/BF00708734
Bouhadiba A, Belhocine Y, Rahim M, Djilani I, Nouar L, Khatmi DE. Host-guest interaction between tyrosine and β-cyclodextrin: Molecular modeling and nuclear studies. J Mol Liq. 2017;233:358–363. doi:10.1016/j.molliq.2017.03.029
Fifere A, Marangoci N, Maier SS, Coroaba A, Maftei D, Pinteala M. Theoretical study on β-cyclodextrin inclusion complexes with propiconazole and protonated propiconazole. Beilstein J Org Chem. 2012;8:2191–2201. doi:10.3762/bjoc.8.247
Mazurek AH, Szeleszczuk Ł. Current status of quantum chemical studies of cyclodextrin host–guest Complexes. Molecules 2022;27:3874. doi:10.3390/molecules27123874
Mesri N, Belhocine Y, Messikh N, Sayede A, Mouffok B. Molecular DFT investigation on the inclusion complexation of Benzo[a]pyrene with γ-Cyclodextrin. Macroheterocycles 2021;14(2):164–170. doi:10.6060/mhc210337m
Oqmhula K, Hongo K, Maezono R, Ichibha T. Ab Initio evaluation of complexation energies for cyclodextrin-drug inclusion complexes. ACS Omega 2020;5:19371–19376. doi:10.1021/acsomega.0c01059
Hanna K, de Brauer C, Germain P. Cyclodextrin-enhanced solubilization of pentachlorophenol in water. J Environ Manag. 2004;71(1):1–8. doi:10.1016/j.jenvman.2004.01.001
Neese F. The ORCA program system. Wiley Interdiscip. Rev. Comput Mol Sci. 2012;2:73–78. doi:10.1002/wcms.81
Neese F. Software update: the ORCA program system, version 4.0. WIREs. Comput Mol Sci. 2017;8:e1327. doi:10.1002/wcms.1327
Becke AD. Density-functional exchange-energy approximation with correct asymptotic behavior. Phys Rev A. 1988;38:3098–3100. doi:10.1103/PhysRevA.38.3098
Lee C, Yang W, Parr RG. Development of the Colle-Salvetti correlation-energy formula into a functional of the electron density. Phys Rev B. 1988;37:785–789. doi:10.1103/PhysRevB.37.785
Caldeweyher E, Ehlert S, Hansen A, Neugebauer H, Spicher S, Bannwarth C, Grimme S. A generally applicable atomic-charge dependent London dispersion correction. J Chem Phys. 2019;150:154122. doi:10.1063/1.5090222
Belhocine Y, Rahali S, Allal H, Assaba IM, Ghoniem MG, Ali FAM. A dispersion corrected DFT investigation of the inclusion complexation of dexamethasone with β-Cyclodextrin and molecular docking study of its potential activity against COVID-19. Molec. 2021;26:7622. doi:10.3390/molecules26247622
Litim A, Belhocine Y, Benlecheb T, Ghoniem MG, Kabouche Z, Ali FAM, Abdulkhair BY, Seydou M, Rahali S. DFT-D4 Insight into the Inclusion of Amphetamine and Methamphetamine in Cucurbit[7]uril: Energetic, Structural and Biosensing Properties. Molec. 2021;26:7479. doi:10.3390/molecules26247479
Kruse H, Grimme S. A geometrical correction for the inter-and intra-molecular basis set superposition error in Hartree-Fock and density functional theory calculations for large systems. J Chem Phys. 2012;136:154101. doi:10.1063/1.3700154
Liu L, Guo QX. Use of quantum chemical methods to study cyclodextrin chemistry. J Incl Phenom Macrocycl Chem. 2004;50:95–103. doi:10.1007/s10847-003-8847-3
Jmol: an open-source Java viewer for chemical structures in 3D. Available from: http://www.jmol.org/
Grimme S, Brandenburg JG, Bannwarth C, Hansen A. Consistent structures and interactions by density functional theory with small atomic orbital basis sets. J Chem Phys. 2015;143:054107. doi:10.1063/1.4927476
Marenich AV, Cramer CJ, Truhlar DG. Universal Solvation Model Based on Solute Electron Density and on a Continuum Model of the Solvent Defined by the Bulk Dielectric Constant and Atomic Surface Tensions. J Phys Chem B 2009;113(18):6378–6396. doi:10.1021/jp810292n
Lefebvre C, Khartabil H, Boisson JC, Contreras-García J, Piquemal JP, Hénon E. The Independent Gradient Model: A New Approach for Probing Strong and Weak Interactions in Molecules from Wave Function Calculations. ChemPhysChem 2018;19:724–735. doi:10.1002/cphc.201701325
Lu T, Chen Q. Independent gradient model based on Hirshfeld partition: A new method for visual study of interactions in chemical systems. J Comput Chem. 2022;43:539–555. doi:10.1002/jcc.26812
Lu T, Chen F. Multiwfn: a multifunctional wavefunction analyzer. J Comput Chem. 2012;33:580–592. doi:10.1002/jcc.22885
Humphrey W, Dalke A, Schulten K. VMD: visual molecular dynamics. J Mol Graph. 1996;14:33–38. doi:10.1016/0263-7855(96)00018-5
Knizia G. Intrinsic atomic orbitals: An unbiased bridge between quantum theory and chemical concepts. J Chem Theory Comput. 2013;9(11):4834–4843. doi:10.1021/ct400687b
Knizia G, Klein JE. Electron flow in reaction mechanisms—Revealed from first principles. Angew Chem Int Ed. 2015;54(18):5518–5522. doi:10.1002/anie.201410637
González GB, Espinoza JM. Thermodynamic and reactivity aspect of β-cyclodextrine inclusion complexes with coumarin derivatives. J Chil Chem Soc. 2022;67(2):5514–5520. doi:10.4067/S0717-97072022000205514
Belhocine Y, Bouhadiba A, Rahim M, Nouar L, Djilani I, Khatmi DE. Inclusion complex formation of β-Cyclodextrin with the nonsteroidal anti-inflammatory drug flufenamic acid: computational study. Macroheterocycles. 2018;11(2):203–209. doi:10.6060/mhc170829b
Reed AE, Curtiss LA, Weinhold F. Intermolecular interactions from a natural bond orbital, donor-acceptor viewpoint. Chem Rev. 1998;88:899–926. doi:10.1021/cr00088a005
Zhao Y, Truhlar DG. The M06 suite of density functionals for main group thermochemistry, thermochemical kinetics, noncovalent interactions, excited states, and transition elements: two new functionals and systematic testing of four M06-class functionals and 12 other functionals. Theor Chem Account. 2008;120:215–241. doi:10.1007/s00214-007-0310-x
Weigend F. Accurate Coulomb-fitting basis sets for H to Rn. Phys Chem Chem Phys. 2006;8:1057–1065. doi:10.1039/B515623H
Weigend F, Ahlrichs R. Balanced basis sets of split valence, triple zeta valence and quadruple zeta valence quality for H to Rn: Design and assessment of accuracy. Phys Chem Chem Phys. 2005;7:3297–3305. doi:10.1039/B508541A
Frisch MJ, Trucks GW, Schlegel HB, Scuseria GE, Robb MA, Cheeseman JR, et al. Gaussian 09, Revision D.01, Gaussian Inc., Wallingford (CT), 2013.
DOI: https://doi.org/10.15826/chimtech.2023.10.2.09
Copyright (c) 2023 Zoubir Kabouche, Youghourta Belhocine, Tahar Benlecheb, Ibtissem Meriem Assaba, Abdelkarim Litim, Rabab Lalalou, Asma Mechhoud

This work is licensed under a Creative Commons Attribution 4.0 International License.
© Website Chimica Techno Acta, 2014–2024
ISSN 2411-1414 (Online)
This journal is licensed under a Creative Commons Attribution 4.0 International