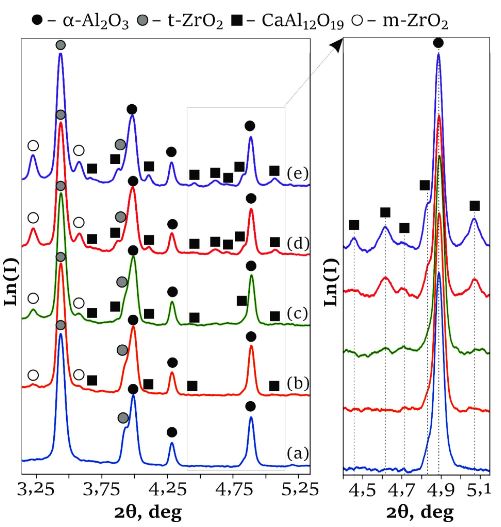
Features of calcium hexaaluminate formation in alumina-zirconia ceramics
Abstract
Keywords
Full Text:
PDFReferences
Yıldız BK, Tür YK. Effect of ZrO2 content on the microstructure and flexural strength of Al2O3–ZrO2 composites with the stored failure energy-fragmentation relations. Ceram Int. 2021;47(24):34199–34206. doi:10.1016/j.ceramint.2021.08.329
Anjaneyulu B, Nagamalleswara Rao G, Prahlada Rao K. Development, mechanical and tribological characterization of Al2O3 reinforced ZrO2 ceramic composites. Mater Today Proc. 2021;37:584–591. doi:10.1016/j.matpr.2020.05.594
Smuk B, Szutkowska M, Walter J. Alumina ceramics with partially stabilized zirconia for cutting tools. J Mater Process Technol. 2003;133(1–2):195–198. doi:10.1016/S0924-0136(02)00232-7
Kern F, Gadow R. In Situ Platelet Reinforcement of Alumina and Zirconia Matrix Nanocomposites – One Concept, Different Reinforcement Mechanisms. Adv Sci Technol. 2014;87:118–125. doi:10.4028/www.scientific.net/AST.87.118
Fornabaio M, Palmero P, Traverso R, Esnouf C, Reveron H, Chevalier J, Montanaro L. Zirconia-based composites for biomedical applications: Role of second phases on composition, microstructure and zirconia transformability. J Eur Ceram Soc. 2015;35(14):4039–4049. doi:10.1016/j.jeurceramsoc.2015.04.027
Piconi C, Maccauro G, Muratori F, Prever EB Del. Alumina and zirconia ceramics in joint replacements. J Appl Biomater Funct Mater. 2003;1(1):19–32. doi:10.1177/228080000300100103
Chen Z, Chawla K., Koopman M. Microstructure and mechanical properties of in situ synthesized alumina/Ba-β-alumina/zirconia composites. Mater Sci Eng A. 2004;367(1–2):24–32. doi:10.1016/j.msea.2003.09.070
Kern F, Gommeringer A. Reinforcement Mechanisms in yttria-ceria-co-stabilized zirconia- alumina-strontium hexaaluminate composite ceramics. J Ceram Sci Technol 2018;98:93–98. doi:10.4416/JCST2017-00046
Zhang X, Liang J, Li J, Zeng Y, Hao S, Liu P, Na H. The properties characterization and strengthening-toughening mechanism of Al2O3-CA6-MA-Ni multi-phase composites prepared by adding calcined dolomite. Mater Charact. 2022;186:111810. doi:10.1016/J.MATCHAR.2022.111810
Sirotinkin V, Podzorova L, Il’icheva A. Comparative X-ray diffraction study of the Yb2O3 stabilized zirconia ceramics doped with SrO and CaO. Mater Chem Phys. 2022;277:125496. doi:10.1016/J.MATCHEMPHYS.2021.125496
Cinibulk MK. Hexaluminates as a cleavable fiber–matrix interphase: synthesis, texture development, and phase compatibility. J Eur Ceram Soc. 2000;20(5):569–582. doi:10.1016/S0955-2219(99)00255-1
Tian M, Wang XD, Zhang T. Hexaaluminates: a review of the structure, synthesis and catalytic performance. Catal Sci Technol. 2016;6(7):1984–2004. doi:10.1039/C5CY02077H
Sktani ZDI, Azhar AZA, Ratnam MM, Ahmad ZA. The influence of in-situ formation of hibonite on the properties of zirconia toughened alumina (ZTA) composites. Ceram Int. 2014;40(4):6211–6217. doi:10.1016/j.ceramint.2013.11.076
Nagaoka T, Yasuoka M, Hirao K, Kanzaki S, Yamaoka Y. Effects of CaO addition on sintering and mechanical properties of Al2O3. J Mater Sci Lett. 1996;15(20):1815–1817. doi:10.1007/BF00275351
Asmi D, Low IM, O’Connor BH. Physical, thermal, and mechanical properties of Al2O3-CaAl12O19 composites processed by in-situ reaction sintering. J Sains MIPA Univ Lampung. 2012;4(1):1–8.
Podzorova LI, Il’icheva AA, Pen’kova OI, Antonova OS, Baikin AS, Konovalov AA. Al2O3-based ceramic composites with a high brittle fracture resistance. Inorg Mater. 2019;55(6):628–33. doi:10.1134/S0020168519060128
Ismail H, Mohamad H. Effects of CaCO3 additive on the phase, physical, mechanical, and microstructural properties of zirconia-toughened alumina-CeO2-Nb2O5 ceramics. Ceram Int. 2023;49(22):36850–36856. doi:10.1016/j.ceramint.2023.09.015
Burger W, Richter HG. High Strength and Toughness Alumina Matrix Composites by Transformation Toughening and «In Situ» Platelet Reinforcement (ZPTA) – The New Generation of Bioceramics. Key Eng Mater. 2000;192–195:545–548. doi:10.4028/www.scientific.net/KEM.192-195.545
Naga SM, Elshaer M, Awaad M, Amer AA. Strontium hexaaluminate/ZTA composites: Preparation and characterization. Mater Chem Phys. 2019;232:23–27. doi:10.1016/j.matchemphys.2019.04.055
Arab A, Ahmad R, Ahmad ZA. Effect of SrCO3 addition on the dynamic compressive strength of ZTA. Int J Miner Metall Mater. 2016;23(4):481–489. doi:10.1007/s12613-016-1259-3
Vishista K, Gnanam FD. Effect of strontia on the densification and mechanical properties of sol–gel alumina. Ceram Int. 2006;32(8):917–922. doi:10.1016/J.CERAMINT.2005.06.014
Podzorova LI, Il’icheva AA, Sirotinkin VP, Antonova OS, Baikin AS, Kutuzova VE, Pen’kova OI. Ceramic composites of the zirconium dioxide and aluminum oxide system including strontium hexaaluminate. Glas Ceram (English Transl Steklo i Keramika). 2021;78(5–6):231–236. doi:10.1007/S10717-021-00385-X
Shi S, Cho S, Goto T, Sekino T. Role of CeAl11O18 in reinforcing Al2O3/Ti composites by adding CeO2. Int J Appl Ceram Technol. 2021;18(1):170–181. doi:10.1111/ijac.13629
Liu M, Wang Z, Luan X, Wu J, Li Q. Effects of CeO2 and Y2O3 on the interfacial diffusion of Ti/Al2O3 composites. J Alloys Compd. 2016;656:929–935. doi:10.1016/j.jallcom.2015.10.043
Roduit N. JMicroVision: Image analysis toolbox for measuring and quantifying components of high-definition images. Version 1.3.4.
Niihara K, Morena R, Hasselman DPH. Evaluation of KIc of brittle solids by the indentation method with low crack-to-indent ratios. J Mater Sci Lett. 1982;1(1):13–16. doi:10.1007/BF00724706
Cui S, Wang Q, Zhou Y, Mao D, Bao J, Song X. Effect of nickel oxide and titanium oxide on the microstructural, optical, and mechanical properties of calcium hexaaluminate ceramics. Ceram Int. 2021;47(24):35302–35311. doi:10.1016/j.ceramint.2021.09.073
Cherkasova NY, Bataev AA, Veselov SV, Kuzmin RI, Stukacheva NS, Zimogliadova TA. Structure and fracture toughness of ceramics based on Al2O3 and ZrO2 with SrAl12O19 additive. Lett Mater. 2019;9(2). doi:10.22226/2410-3535-2019-2-179-184
Suzuki Y, Nishihashi K. Microstructures and mechanical properties of reactively sintered CaAl12O19/CaAl4O7 porous composites. Ceram Int. 2023;49(18):29427–29432. doi:10.1016/j.ceramint.2023.06.004
Zhang X, Zeng Y, Liang J, Li J, Hao S, Wang Y. The microstructure and mechanical properties of Ni/Al2O3 composites by in-situ generated CaAl12O19 and ZrO2 via hot pressing sintering. Ceram Int. 2020;46(9):13144–13150. doi:10.1016/j.ceramint.2020.02.088
Gogotsi GA. Fracture toughness of ceramics and ceramic composites. Ceram Int. 2003;29(7):777–784. doi:10.1016/S0272-8842(02)00230-4
Evans AG, Charles EA. Fracture toughness determinations by indentation. J Am Ceram Soc. 1976;59(7–8):371–372. doi:10.1111/j.1151-2916.1976.tb10991.x
Cherkasova N, Kuzmin R, Veselov S, Antropova K, Ruktuev A, Ogneva T, Tyurin A, Kuchumova I, Khabirov R. Influence of strontium hexaaluminate percentage on the structure and properties of alumina-zirconia ceramics. Mater Chem Phys. 2022;288:126424. doi:10.1016/j.matchemphys.2022.126424
Cherkasova NY, Kuz’min RI, Antropova KA, Burkhinova NY. Rheological characteristics of suspensions and structure of Al2O3–CaO and Al2O3–SrO composites. Refract Ind Ceram. 2022;63(3):311–314. doi:10.1007/s11148-022-00727-4
DOI: https://doi.org/10.15826/chimtech.2023.10.3.17
Copyright (c) 2023 Nina Cherkasova, Kristina Antropova, Ruslan Kuzmin, Kemal Emurlaev, Ivanna Kuchumova, Nomina Burkhinova, Yulia Zobova

This work is licensed under a Creative Commons Attribution 4.0 International License.
© Website Chimica Techno Acta, 2014–2024
ISSN 2411-1414 (Online)
This journal is licensed under a Creative Commons Attribution 4.0 International