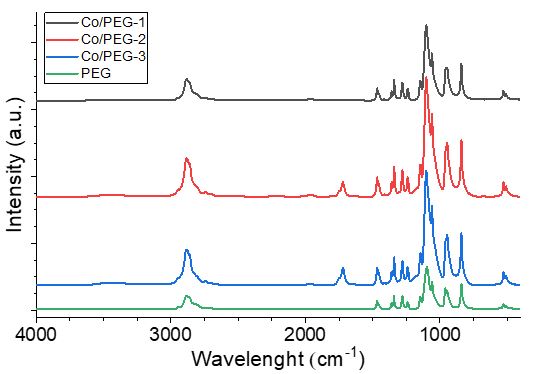
Towards controlling the morphology of cobalt loaded nanocomposites in polyol process with polyethylene glycol
Abstract
The polyol process is one of the simple, efficient and productive methods for the synthesis of metal loaded polymer composites. Functional properties of metal-polymer nanocomposites are determined by chemical composition, size and morphology of their particles. Finding effective ways to control the nanoparticle's properties during the polyol process is a crucial task. The effect of molar ratio Mn+/OHPEG on the formation of cobalt loaded metal-polymer nanocomposites during a one-pot two-component polyol process by polyethylene glycol with Mr = 4000 g·mol–1 (PEG) was studied. The PEG-based polyol process and the formation of cobalt nanophase were studied at molar ratios νCo2+/νOH(PEG) = 1:1, 1:10, 1:100 and 1:500 using UV-Vis, diffuse reflectance IR and ATR FT-IR spectroscopy, nanoparticle tracking analysis (NTA), dynamic light scattering (DLS). It was found that PEG can act as a reducing agent and stabilizing matrix for the cobalt nanophase at a ratio higher than Mn+/OHPEG= 1:10. The composition and morphology of Co/PEG nanocomposites were determined by XRD and TEM methods. Two types of spheroid particles with average diameters of 88±55 nm / 8±4 nm and 12±3 nm / 3±1 nm, respectively, represent Co/PEG nanocomposites 1:500 and 1:100. Scaly structures with a diameter of 15±5 nm are formed at a molar ratio of νCo2+/νOH(PEG) = 1:10. An increase in the Co2+ content in the PEG-based polyol process leads to the immobilized cobalt nanophase Co3O4 (1:500), Co0/CoO (1:100), CoO (1:10) in PEG. Co/PEG nanocomposites are hemocompatible. The HC50value depends on the composition and morphology of the nanoparticles.
Keywords
Full Text:
PDFReferences
Kharisov BI, Dias HVR, Kharissova OV. Mini-review: Ferrite nanoparticles in the catalysis. Arab J Chem. 2019;12(7):1234–1246. doi:10.1016/j.arabjc.2014.10.049
Matsui I. Nanoparticles for electronic device applications: a brief review. J Chem Eng Japan. 2005;38(8):535–546. doi:10.1252/jcej.38.535
Mauricio MD, Guerra-Ojeda S, Marchio P, et al. Nanoparti-cles in Medicine: a focus on vascular oxidative stress. Oxi-dative Med Cellular Longevity. 2018;2018:1–20. doi:10.1155/2018/6231482
Zhang L, Gu F, Chan J, Wang A, Langer R, Farokhzad O. Na-noparticles in medicine: therapeutic applications and de-velopments. Clin Pharmacol Ther. 2008;83(5):761–769. doi:10.1038/sj.clpt.6100400
Rezić I. Nanoparticles for Biomedical application and their synthesis. Polymers. 2022;14(22):4961. doi:10.3390/polym14224961
Ammar S, Fiévet F. Polyol synthesis: a versatile wet-chemistry route for the design and production of functional inorganic nanoparticles. Nanomater. 2020;10(6):1217. doi:10.3390/nano10061217
Ruz P, Sudarsan V. Polyol Method for synthesis of nano-materials. In: Tyagi AK, Ningthoujam RS, eds. Handbook on Synthesis Strategies for Advanced Materials. Indian Insti-tute of Metals Series. Springer Singapore; 2021:293–332. doi:10.1007/978-981-16-1807-9_11
Dong H, Chen YC, Feldmann C. Polyol synthesis of nanopar-ticles: status and options regarding metals, oxides, chalco-genides, and non-metal elements. Green Chem. 2015;17(8):4107–4132. doi:10.1039/C5GC00943J
Li CC, Zeng HC. Cobalt (hcp) nanofibers with pine-tree-leaf hierarchical superstructures. J Mater Chem. 2010;20(41):9187. doi:10.1039/c0jm01621g
Park BK, Jeong S, Kim D, Moon J, Lim S, Kim JS. Synthesis and size control of monodisperse copper nanoparticles by polyol method. J Colloid Interface Sci. 2007;311(2):417–424. doi:10.1016/j.jcis.2007.03.039
Soumare Y, Garcia C, Maurer T, et al. Kinetically controlled synthesis of hexagonally close‐packed cobalt nanorods with high magnetic coercivity. Adv Funct Mater. 2009;19(12):1971–1977. doi:10.1002/adfm.200800822
Gautam A, Singh GP, Ram S. A simple polyol synthesis of silver metal nanopowder of uniform particles. Synthetic Metals. 2007;157(1):5–10. doi:10.1016/j.synthmet.2006.11.009
Burmatova A, Khannanov A, Gerasimov A, et al. A hyper-branched polyol process for designing and manufacturing nontoxic cobalt nanocomposite. Polymers. 2023;15(15):3248. doi:10.3390/polym15153248
Navaladian S, Viswanathan B, Viswanath RP, Varadarajan TK. Thermal decomposition as route for silver nanoparti-cles. Nanoscale Res Lett. 2007;2(1):44. doi:10.1007/s11671-006-9028-2
Izu N, Matsubara I, Uchida T, Itoh T, Shin W. Synthesis of spherical cobalt oxide nanoparticles by a polyol method. J Ceram Soc Japan. 2017;125(9):701–704. doi:10.2109/jcersj2.17114
Logutenko OA, Titkov AI, Vorob’yov AM, et al. Effect of mo-lecular weight of sodium polyacrylates on the size and morphology of nickel nanoparticles synthesized by the modified polyol method and their magnetic properties. Eur Polymer J. 2018;99:102–110. doi:10.1016/j.eurpolymj.2017.12.017
Zhang X, Yin H, Cheng X, Jiang Z, Zhao X, Wang A. Modify-ing effects of polyethylene glycols and sodium dodecyl sul-fate on synthesis of Ni nanocrystals in 1,2-propanediol. Appl Surface Sci. 2006;252(23):8067–8072. doi:10.1016/j.apsusc.2005.10.010
Stiufiuc R, Iacovita C, Nicoara R, et al. One-Step synthesis of pegylated gold nanoparticles with tunable surface charge. J Nanomater. 2013;2013:1-7. doi:10.1155/2013/146031
Stiufiuc R, Iacovita C, Lucaciu CM, et al. SERS-active silver colloids prepared by reduction of silver nitrate with short-chain polyethylene glycol. Nanoscale Res Lett. 2013;8(1):47. doi:10.1186/1556-276X-8-47
Díaz-Cruz C, Alonso Nuñez G, Espinoza-Gómez H, Flores-López LZ. Effect of molecular weight of PEG or PVA as re-ducing-stabilizing agent in the green synthesis of silver-nanoparticles. Eur Polymer J. 2016;83:265–277. doi:10.1016/j.eurpolymj.2016.08.025
Iacovita C, Stiufiuc R, Radu T, et al. Polyethylene glycol-mediated synthesis of cubic iron oxide nanoparticles with high heating power. Nanoscale Res Lett. 2015;10(1):391. doi:10.1186/s11671-015-1091-0
Haddad PS, Santos MC, De Guzzi Cassago CA, Bernardes JS, De Jesus MB, Seabra AB. Synthesis, characterization, and cytotoxicity of glutathione-PEG-iron oxide magnetic nano-particles. J Nanopart Res. 2016;18(12):369. doi:10.1007/s11051-016-3680-y
Abbas M, Nazrul Islam Md, Parvatheeswara Rao B, Ogawa T, Takahashi M, Kim C. One-pot synthesis of high magnetiza-tion air-stable FeCo nanoparticles by modified polyol method. Mater Lett. 2013;91:326–329. doi:10.1016/j.matlet.2012.10.019
Banerjee SS, Aher N, Patil R, Khandare J. Poly(ethylene glycol)-prodrug conjugates: concept, design, and applica-tions. J Drug Deliv. 2012;2012:1-17. doi:10.1155/2012/103973
Lyseng-Williamson KA. Macrogol (polyethylene glycol) 4000 without electrolytes in the symptomatic treatment of chronic constipation: a profile of its use. Drugs Ther Per-spect. 2018;34(7):300–310. doi:10.1007/s40267-018-0532-0
Aoun N, Schlange A, Dos Santos AR, Kunz U, Turek T. Effect of the OH−/Pt ratio during polyol synthesis on metal load-ing and particle size in DMFC catalysts. Electrocatal. 2016;7(1):13–21. doi:10.1007/s12678-015-0275-9
Khannanov A, Burmatova A, Ignatyeva K, et al. Effect of the synthetic approach on the formation and magnetic proper-ties of iron-based nanophase in branched polyester polyol matrix. IJMS. 2022;23(23):14764. doi:10.3390/ijms232314764
Khannanov A, Rossova A, Ulakhovich N, et al. Doxorubicin-loaded hybrid micelles based on carboxyl-terminated hy-perbranched polyester polyol. ACS Appl Polym Mater. 2022;4(4):2553–2561. doi:10.1021/acsapm.1c01863
Malloy A, Hole P, Carr B. Nanoparticle tracking analysis; the halo system. MRS Proc. 2006;952:0952-F02-04. doi:10.1557/PROC-0952-F02-04
Kashkanova AD, Blessing M, Gemeinhardt A, Soulat D, San-doghdar V. Precision size and refractive index analysis of weakly scattering nanoparticles in polydispersions. Nat Methods. 2022;19(5):586–593. doi:10.1038/s41592-022-01460-z
Dragovic RA, Gardiner C, Brooks AS, et al. Sizing and phe-notyping of cellular vesicles using nanoparticle tracking analysis. Nanomed Nanotechnol Biol Med. 2011;7(6):780–788. doi:10.1016/j.nano.2011.04.003
Klajnert B, Walach W, Bryszewska M, Dworak A, Shcharbin D. Cytotoxicity, haematotoxicity and genotoxicity of high molecular mass arborescent polyoxyethylene polymers with polyglycidol‐block‐containing shells. Cell Biol Int. 2006;30(3):248–252. doi:10.1016/j.cellbi.2005.10.026
Parveen S, Sahoo SK. Nanomedicine: clinical applications of polyethylene glycol conjugated proteins and drugs. Clin Pharmacokinet. 2006;45(10):965–988. doi:10.2165/00003088-200645100-00002
Ren J, Lin T, Sprague LW, Peng I, Wang LQ. Exploring chemical equilibrium for alcohol-based cobalt complexa-tion through visualization of color change and UV–vis spec-troscopy. J Chem Educ. 2020;97(2):509–516. doi:10.1021/acs.jchemed.9b00264
Aranishi K, Zhu Q, Xu Q. Dendrimer‐encapsulated cobalt nanoparticles as high‐performance catalysts for the hy-drolysis of ammonia borane. ChemCatChem. 2014;6(5):1375–1379. doi:10.1002/cctc.201301006
Imadadulla M, Nemakal M, Koodlur Sannegowda L. Solvent dependent dispersion behaviour of macrocycle stabilized cobalt nanoparticles and their applications. New J Chem. 2018;42(14):11364–11372. doi:10.1039/C8NJ01773E
Alrehaily LM, Joseph JM, Biesinger MC, Guzonas DA, Wren JC. Gamma-radiolysis-assisted cobalt oxide nanoparticle formation. Phys Chem Chem Phys. 2013;15(3):1014–1024. doi:10.1039/C2CP43094K
Singh J, Tripathi J, Kaurav N. Synthesis and characteriza-tion of Co nanoparticles. AIP Conf Proc. 2017:050083. doi:10.1063/1.4980316
Spiridonov VV, Sybachin AV, Pigareva VA, et al. One-step low temperature synthesis of CeO2 nanoparticles stabilized by carboxymethylcellulose. Polymers. 2023;15(6):1437. doi:10.3390/polym15061437
DOI: https://doi.org/10.15826/chimtech.2023.10.4.13
Copyright (c) 2023 Anastasia Burmatova, Artur Khannanov, Liana Zubaidullina, Dmitry Emelianov, Olga Mostovaya, Ivan Stoikov, Nikolay Ulakhovich, Marianna Kutyreva

This work is licensed under a Creative Commons Attribution 4.0 International License.
Chimica Techno Acta, 2014-2024
ISSN 2411-1414 (Online)
Copyright Notice