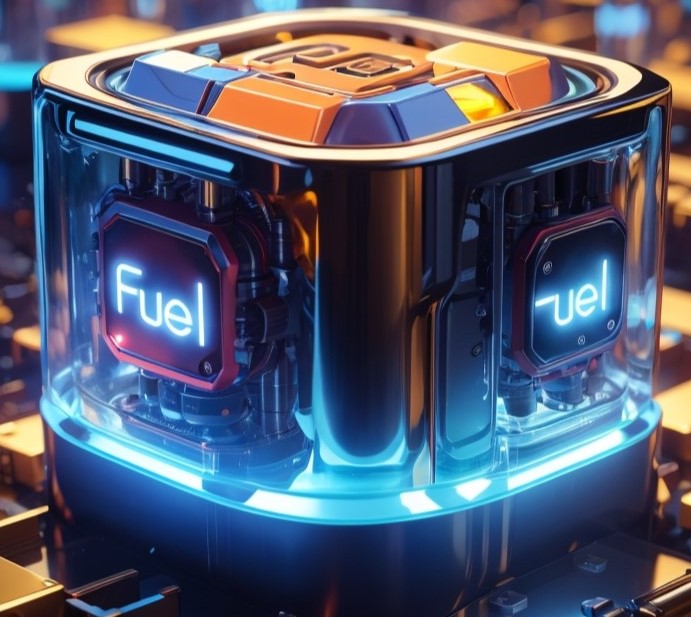
Solid state electrochemical devices for hydrogen energy
Abstract
The idea of nuclear-hydrogen energy draws growing interest of power engineering specialists. One of the ways to implement such a direction of development is integration of nuclear power station and high temperature electrochemical devices, namely solid oxide electrolyzers (SOE) and fuel cells (SOFC). This paper reviews the principal features of the devices and their high efficiency of energy transformation. The electrical efficiency of the SOEs can exceed 100 % at the expense of high-grade potential heat energy consumed by the electrolyzer. The efficiency of the SOFCs amounts to 70 %. The state of the art of long-term tests of single cells of SOEs and SOFC stacks are presented.
Keywords
Full Text:
PDFReferences
Legasov VA, Ponomarev-Stepnoy NN, Protsenko AN, Chernilin YuF et al., Atomno-vodorodnaya energetika (prognoz razvitiya) [Nuclear-hydrogen energy (development forecast)], Questions of Nuclear Science and Technology (Nuclear-Hydrogen Energy) 1 (1976) 5–34 (in Russian).
Ponomarev-Stepnoy NN, Stolyarevskii AYa, Pakhomov VP, Atomno-vodorodnaya energetika [Nuclear-Hydrogen Energy], Nuclear Energy 96 (6) (2004) 411–425 (in Russian). Available from: http://elib.biblioatom.ru/text/atomnaya-energiya_t96-6_2004/go,3/, Accessed on 14 June 2023.
Graves C, Ebbesen SD, Mogensen M, Lackner KS, Sustainable hydrocarbon fuels by recycling CO2 and H2O with renewable or nuclear energy, Renewable Sustain. Energy Rev. 15 (1) (2011) 1–23. https://doi.org/10.1016/j.rser.2010.07.014
Perfiliev MV, Demin AK, Kuzin BL, Lipilin AS, Vysokotemperaturnii electroliz gazov [High-Temperature Gas Electrolysis]. Nauka: Moscow; 1988. 232 p. (in Russian).
Laguna-Bercero MA, Recent advances in high temperature electrolysis using solid oxide fuel cells: A review, J. Power Sources 203 (2012) 4–16. https://doi.org/10.1016/j.jpowsour.2011.12.019
Hino R, Haga K, Aita H, Sekita K, R&D on hydrogen production by high-temperature electrolysis of steam, Nuclear Eng. Design 233 (1–3) (2004) 363–375. https://doi.org/10.1016/j.nucengdes.2004.08.029
Forsberg C, Futures for hydrogen produced using nuclear energy, Progress in Nuclear Energy 47 (1–4) (2005) 484–495. https://doi.org/10.1016/j.pnucene.2005.05.049
Utgikar V, Thiesen T, Life cycle assessment of high temperature electrolysis for hydrogen production via nuclear energy, Int. J. Hydrogen Energy 31 (7) (2006) 939–944. https://doi.org/10.1016/j.ijhydene.2005.07.001
Harvego EA, McKellar MG, O’Brien JE, Herring JS, Parametric evaluation of large-scale high-temperature electrolysis hydrogen production using different advanced nuclear reactor heat sources, Nucl. Eng. Design 239 (9) (2009) 1571–1580. https://doi.org/10.1016/j.nucengdes.2009.03.003
Stoots CM, O’Brien JE, Condie KG, Hartvigsen JJ, High-temperature electrolysis for large-scale hydrogen production from nuclear energy - experimental investigations, Int. J. Hydrogen Energy 35 (10) (2010) 4861–4870. https://doi.org/10.1016/j.ijhydene.2009.10.045
O’Brien JE. Large scale hydrogen production from nuclear energy using high temperature electrolysis. In: Proc. 14th International Heat Transfer Conference; 2010 August 3–18; Washington D.C., USA. 23341.
Zhang W, Yu B, Xu J, Efficiency evaluation of high-temperature steam electrolytic systems coupled with different nuclear reactors, Intern. J. Hydrogen Energy 37 (17) (2012) 12060–12068. https://doi.org/10.1016/j.ijhydene.2012.04.024
Giraldi MR, Francois J-L, Martin-del-Campo C, Life cycle assessment of hydrogen production from a high temperature electrolysis process coupled to a high temperature gas nuclear reactor, Intern. J. Hydrogen Energy 40 (10) (2015) 4019–4033. https://doi.org/10.1016/j.ijhydene.2015.01.093
El-Emam RS, Khamis I, International collaboration in the IAEA nuclear hydrogen production program for benchmarking of HEEP, Intern J. Hydrogen Energy 42 (6) (2017) 3566–3571. https://doi.org/10.1016/j.ijhydene.2016.07.256
Milewski J, Kupecki J, Szczęśniak A, Uzunow N, Hydrogen production in solid oxide electrolyzers coupled with nuclear reactors, Intern. J Hydrogen Energy 46 (72) (2021) 35765–35776. https://doi.org/10.1016/j.ijhydene.2020.11.217
Calipse F, Cappiello FL, Cimmino L, Vicidomini M, Dynamic simulation modeling of reversible solid oxide fuel cells for energy storage purpose, Energy 260 (2022) 124893. https://doi.org/10.1016/j.energy.2022.124893
Koh JH, Yoon DJ, Oh CH, Simple electrolyzer model development for high-temperature electrolysis system analysis using solid oxide electrolysis cell, J. Nucl. Sci. Techn. 47 (7) (2010) 599–607. Available from: https://www.tandfonline.com/doi/abs/10.1080/18811248.2010.9720957, Accessed on 14 June 2023.
Minh NQ, Mogensen MB, Reversible solid oxide fuel cell technology for green fuel and power production, Interface 22 (4) (2013) 55–62. https://doi.org/10.1149/2.F05134if
Di Giorgio P, Desideri U, Potential of reversible solid oxide cells as electricity storage system, Energies 9 (8) (2016) 662. https://doi.org/10.3390/en9080662
Osinkin DA, Bogdanovich NM, Beresnev SM, Pikalova EYu et al., Reversible solid oxide fuel cell for power accumulation and generation, Russian J. Electrochem. 54 (8) (2018) 644–649. https://doi.org/10.1134/S1023193518080050
Venkataraman V, Pérez-Fortes M, Wang L, Hajimolana YS et al., Reversible solid oxide systems for energy and chemical applications – Review & perspectives, J. Energy Storage 24 (2019) 100782. https://doi.org/10.1016/j.est.2019.100782
Zheng W, Zhang M, Li Y, Shao Z, Wang X, Optimal dispatch for reversible solid oxide cell-based hydrogen/electric vehicle aggregator via stimuli-responsive charging decision estimation, Intern. J. Hydrogen Energy 47 (13) (2022) 8502–8513. https://doi.org/10.1016/j.ijhydene.2021.12.157
Graves C, Ebbesen SD, Jensen SH, Simonsen SB, Mogensen MB, Reversible SOFC degradation eliminating degradation in solid oxide electrochemical cells by reversible operation, Nature Materials 14 (2) (2015) 239–244. https://doi.org/10.1038/nmat4165
Yu M, Tong X, Sudireddy BR, Chen M, Performance and durability of reversible solid oxide cells with nano-electrocatalysts infiltrated electrodes, JOM 74 (2022) 4495–4595. https://doi.org/10.1007/s11837-022-05540-5
Sampathkumar SN, Aubin Ph, Couturier K, Sun X, Sudireddy BR et al., Degradation study of a reversible solid oxide cell (rSOC) short stack using distribution of relaxation times (DRT) analysis, Intern. J. Hydrogen Energy 47 (18) (2022) 10175–10193. https://doi.org/10.1016/j.ijhydene.2022.01.104
Yang C, Guo R, Jing X, Li P et al., Degradation mechanism and modeling study on reversible solid oxide cell in dual-mode – A review, J. Hydrogen Energy 47 (89) (2022) 37895–37928. https://doi.org/10.1016/j.ijhydene.2022.08.240
Buttler A, Spliethoff H. Current status of water electrolysis for energy storage, grid balancing and sector coupling via power-to-gas and power-to-liquids: A review, Renewable Sustain. Energy Rev. 82 (3) (2018) 2440–2454. https://doi.org/10.1016/j.rser.2017.09.003
David M, Ocampo-Martinez C, Sánchez-Peña R, Advances in alkaline water electrolyzers: A review, J. Energy Storage 23 (2019) 392–403. https://doi.org/10.1016/j.est.2019.03.001
Anghilante R, Colomar D, Brisse A, Marrony M, Bottom-up cost evaluation of SOEC systems in the range of 10–100 MW, Intern J Hydrogen Energy 43 (45) (2018) 20309–20322. https://doi.org/10.1016/j.ijhydene.2018.08.161
Schefold J, Brisse A, Poepke H, 23,000 h steam electrolysis with an electrolyte supported solid oxide cell, Intern. J. Hydrogen Energy 42 (19) (2017) 13415–13426. https://doi.org/10.1016/j.ijhydene.2017.01.072
Schefold J, Poepke H, Brisse A, Solid oxide electrolyser cell testing up to the above 30,000 h time range, ECS Trans. 97 (7) (2020) 553–563. https://doi.org/10.1149/MA2020-01361451mtgabs
Fang Q, Blum L, Stolten D, Electrochemical performance and degradation analysis of an SOFC short stack following operation of more than 100,000 hours, ECS Transactions 91 (1) (2019) 687–696. https://doi.org/10.1149/09101.0687ecst
Groß-Barsnick SM, Fang Q, Batfalsky P, Niewolak L, Blum L, Quadakkers WJ, Post-test characterization of metallic materials and adjacent components in an SOFC stack after 34,000 h operation at 700 °C, Fuel Cells 19 (1) (2019) 84–95. https://doi.org/10.1002/fuce.201800050
Kasyanova AV, Zvonareva IA, Tarasova NA, Bi L, et al., Electrolyte materials for protonic ceramic electrochemical cells: Main limitations and potential solutions, Materials Reports: Energy 2 (4) (2022) 100158. https://doi.org/10.1016/j.matre.2022.100158
Heras-Juaristi G, Perez-Coll D, Mather GC, Temperature dependence of partial conductivities of the BaZr0.7Ce0.2Y0.1O3- proton conductor, J. Power Sources 364 (2017) 52–60. https://doi.org/10.1016/j.jpowsour.2017.08.011
Choi S, Davenport TC, Haile SM, Protonic ceramic electrochemical cells for hydrogen production and electricity generation: Exceptional reversibility, stability, and demonstrated faradaic efficiency, Energy Environ. Sci. 12 (2019) 206–215. https://doi.org/10.1039/C8EE02865F
Choi M, Paik J, Kim D, Woo D, et al., Exceptionally high performance of protonic ceramic fuel cells with stoichiometric electrolytes, Energy Environ. Sci. 14 (2021) 6476–6483. https://doi.org/10.1039/D1EE01497H
Demin AK, Tsiakaras PE, Sobyanin VA, Hramova SY, Thermodynamic analysis of a methane fed SOFC system based on a protonic conductor, Solid State Ionics, 152–153 (2002) 555–560. https://doi.org/10.1016/S0167-2738(02)00363-6
DOI: https://doi.org/10.15826/elmattech.2023.2.016
Copyright (c) 2023 Anatoly K. Demin, Dimitry I. Bronin

This work is licensed under a Creative Commons Attribution 4.0 International License.