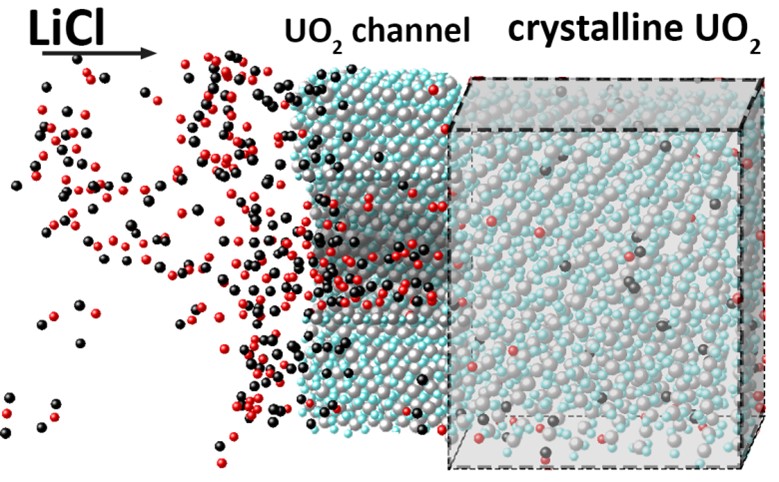
Modeling the UO2 reduction process
Abstract
Methods of molecular dynamics and DFT calculations have been used to study the reduction mechanisms of UO2 as the most representative part of spent nuclear fuel to metallic uranium. It is shown that the critical softening of the combined modulus of elasticity C11-C12 to zero is the reason for the destruction of the UO2 crystal as a result of the removal of oxygen from it. This destruction is accompanied by an order-disorder phase transition in the oxygen subsystem of the crystal under consideration. DFT calculations indicate a continuous decrease in the band gap as oxygen is removed from the UO2 crystal. When the system reaches the composition U2O3, the band gap disappears and the system becomes electrically conductive. The appearance of the dielectric-conductor transition explains the realization of the FFC Cambridge process during the recovery of spent nuclear fuel. The passage of Li+ and Cl– ions of the LiCl melt through cylindrical channels in a UO2 crystal with cross-sectional radii from 0.25 up to 2 nm has been studied. The strength of the external electric field required for the passage of these channels decreases with an increase in the channel cross section, and the number of Cl– ions entering the channel increases. On the walls of the channels that pass ions with charges of both signs, colonies of adsorbed Cl– and Li+ atoms appear separated from each other, between which strong electric fields are formed. The existence of such fields can cause Li+ ions to move deep into the material being reduced.
Keywords
Full Text:
PDFReferences
Galashev AY, Processing of fast neutron reactor fuel by electrorefining: Thematic overview, Int. J. Energy Res. 45 (8) (2021) 11459–11478. https://doi.org/10.1002/er.6267
Herrmann SD, Li SX, Simpson MF, Phongikaroon S, Electrolytic reduction of spent nuclear oxide fuel as part of an integral process to separate and recover actinides from fission products, Separation Sci. Technol. 41 (10) (2006) 1965–1983. https://doi.org/10.1080/01496390600745602
Choi E-Y, Jeong SM, Electrochemical processing of spent nuclear fuels: An overview of oxide reduction in pyroprocessing technology, Prog. Nat. Sci.: Mater. Intern. 25 (6) (2015) 572–582. https://doi.org/10.1016/j.pnsc.2015.11.001
Herrmann S, Li S, Simpson M, Electrolytic reduction of spent light water reactor fuel bench-scale experiment results, J. Nucl. Sci. Technol. 44 (3) (2007) 361–367. https://doi.org/10.1080/18811248.2007.9711295
Kang YH, Hwang SC, Lee HS, et al., Effects of neodymium oxide on an electrorefining process of uranium, J. Mater. Proc. Technol. 209 (11) (2009) 5008–5013. https://doi.org/10.1016/j.jmatprotec.2009.01.024
Galashev AY, Ivanichkina KA, Zaikov YP, Computational study of physical properties of low oxygen UO2–x compounds, J. Solid State Chem. 286 (2020) 121278. https://doi.org/10.1016/j.jssc.2020.121278
Galashev AY, Manzhurov AI, Zaikov YP, Computer modeling of electrochemical processing of waste nuclear fuel, Int. J. Energy Research, 45(8) (2021) 11664–11676. https://doi.org/10.1002/er.5462
Galashev AY, Recovery of actinides and fission products from spent nuclear fuel via electrolytic reduction: Thematic overview, Int. J. Energy Res. 46(4) (2022) 3891–3905. https://doi.org/10.1002/er.7458
Shishkin AV, Shishkin VY, Nikolaev AY, et al., Reduction of ZrO2 in LiCl-Li2O melt during electrolysis, J. Electrochem. Soc. 169 (2022) 116506. https://doi.org/10.1149/1945-7111/ac9f73
Nikolaev A, Suzdaltsev A, Pavlenko O, et al., Reduction of ZrO2 during SNF pyrochemical reprocessing, J. Electrochem. Soc. 168 (2021) 036506. https://doi.org/10.1149/1945-7111/abe8be
Kaloni TP, Onder N, Pencer J, Torres E, DFT + U approach on the electronic and thermal properties of hypostoichiometric UO2, Ann. Nucl. Energy, 144 (2020) 107511. https://doi.org/10.1016/j.anucene.2020.107511
Soler JM, Artacho E, Gale JD, et al., The SIESTA method for ab initio order-N materials simulation, J. Phys.: Condens. Matter, 14 (2002) 2745. https://doi.org/10.1088/0953-8984/14/11/302
Dudarev SL, Botton GA, Savrasov SY, et al., Electron-energy-loss spectra and the structural stability of nickel oxide: An LSDA+U study, Phys. Rev. B, 57 (1998) 1505. https://doi.org/10.1103/PhysRevB.57.1505
Perdew JP, Zunger A, Self-interaction correction to density-functional approximations for many-electron systems, Phys. Rev. B, 23 (1981) 5048. https://doi.org/10.1103/PhysRevB.23.5048
Monkhorst HJ, Pack JD, Special points for Brillouin-zone integrations, Phys. Rev. B, 13 (1976) 5188. https://doi.org/10.1103/PhysRevB.13.5188
Liang T, Shan T-R, Cheng Y-T, et al., Classical atomistic simulations of surfaces and heterogeneous interfaces with the charge-optimized many body (COMB) potentials, Mater. Sci. Eng.: R: Reports, 74 (9) (2013) 255–79. https://doi.org/10.1016/j.mser.2013.07.001
Tersoff J, Modelng solid-state chemistry: Interatomic potentials for multicomponent systems, Phys. Rev. B: Condens. Matter Mater. Phys. 39 (1989) 5566. https://doi.org/10.1103/PhysRevB.39.5566
Phillpot SR, Antony AC, Shi L, et al., Optimized many body (COMB) potentials for simulation of nuclear fuel and clad, Comp. Mater. Sci. 148 (2018) 231–241. https://doi.org/10.1016/j.commatsci.2018.02.041
Nakano A, Parallel multilevel preconditioned conjugate gradient approach to variable-charge molecular dynamics, Comp. Phys. Commun. 104 (1–3) (1997) 59–69. https://doi.org/10.1016/S0010-4655(97)00041-6
Grippo L, Lucidi S, A global convergent version of the Polak-Ribiere conjugate gradient method, Math. Program. 78 (1997) 375–391. https://doi.org/10.1007/BF02614362
Basak CB, Sengupta AK, Kamath HS, Classical molecular dynamics simulation of UO2 to predict thermophysical properties, J. Alloys and Compounds, 360 (1–2) (2003) 210216. https://doi.org/10.1016/S0925-8388(03)00350-5
Tosi MP, Fumi FG, Ionic sizes and born repulsive parameters in the NaCl-type alkali halidesII: The generalized Huggins-Mayer form, J. Phys. Chem. Solids, 25 (1) (1964) 45–52. https://doi.org/10.1016/0022-3697(64)90160-X
Nekrasov KA, Galashev AE. Diffusion of oxygen ions during electrochemical reduction of uranium dioxide in LiCl melt. Molecular dynamics modeling, collection of abstracts. In: Abstracts of the VIII scientific seminar “Modeling of nuclear fuel cycle technologies”; 2019.01.28–2019.02.01, Snezhinsk, Russia. p. 18.
Oda T, Oya Y, Tanaka S, Weber WJ, Validation of potential models for Li2O in classical molecular dynamics simulation, J. Nucl. Mater. 367–370 (2007) 263–268. https://doi.org/10.1016/j.jnucmat.2007.03.139
Olander DR, Nuclear fuels: Present and future, Engineering J. 13 (1) (2009) 1–28. https://doi.org/10.4186/ej.2009.13.1.1
Mieszczynski C, Kuri G, Bertsch J, et al., Microbeam x-ray absorption spectroscopy study of chromium in large-grain uranium dioxide fuel, J. Phys. Condens. Matter, 26 (35) (2014) 355009. https://doi.org/10.1088/0953-8984/26/35/355009
Mowrey DD, Xu L, Mei Y, et al., Ion-pulling simulations provide insights into the mechanisms of channel opening of the skeletal muscle ryanodine receptor, J. Biol. Chem. 292 (31) (2017) 12947–12958. https://doi.org/10.1074/jbc.M116.760199
Mouhat F, Coudert F-X, Necessary and sufficient elastic stability conditions in various crystal systems, Phys. Rev. B, 90 (2014) 224104. https://doi.org/10.1103/PhysRevB.90.224104
Dugan CL, Peterson GG, Mock A, et al., Electrical and material properties of hydrothermally grown single crystal (111) UO2, Eur. Phys. J. B, 91 (2018) 67. https://doi.org/10.1140/epjb/e2018-80489-x
Prodan ID, Scuseria GE, Martin RL, Assessment of metageneralized gradient approximation and screened Coulomb hybrid density functionals on bulk actinide oxides, Phys. Rev. B. 73 (2006) 045104. https://doi.org/10.1103/PhysRevB.73.045104
Hamad H, Elsenety MM, Sadik W, et al., The superior photocatalytic performance and DFT insights of S scheme CuO@TiO2 heterojunction composites for simultaneous degradation of organics, Sci. Rep. 12 (2022) 2217. https://doi.org/10.1038/s41598-022-05981-7
Farouk T, Farouk B, Staack D, et al., Modeling of direct current micro-plasma discharges in atmospheric pressure hydrogen, Plasma Sources Sci. Technol. 16 (2007) 619–634. https://doi.org/10.1088/0963-0252/16/3/023
Sri Maha Vishnu D, Sanil N, Panneerselvam G, et al., Mechanism of direct electrochemical reduction of solid UO2 to uranium metal in CaCl2-48mol% NaCl melt, J. Electrochem. Soc. 160 (9) (2013) D394. https://doi.org/10.1149/2.080309jes
Chen GZ, Fray DJ, Farthing TW, Direct electrochemical reduction of titanium dioxide to titanium in molten calcium chloride, Nature, 407 (2000) 361–364. https://doi.org/10.1038/35030069
Gruber H, Krautz E, Magnetoresistance and conductivity in the binary-system titanium oxygen. II. Semiconductive titanium-oxides, Phys. Status Solidi A, 69 (1982) 287–295. https://doi.org/10.1002/pssa.2210750222
Chen GZ, Fray DJ, Voltammetric studies of the oxygen-titanium binary system in molten calcium chloride, J. Electrochem. Soc. 149 (11) (2002) E455–E467. https://doi.org/10.1149/1.1513985
Dring K, Dashwood R, Inman D, Voltammetry of titanium dioxide in molten calcium chloride at 900°C. J. Electrochem. Soc. 152 (3) (2005) E104–E113. https://doi.org/10.1149/1.1860515
Moten SA, Atta-Fynn R, Ray AK, Huda MN, Size effects on the electronic and magnetic properties of PuO2 (111) surface, J. Nucl. Mater. 468 (2016) 37–45. https://doi.org/10.1016/j.jnucmat.2015.11.009
Anisimov VI, Zaanen J, Andersen OK, Band theory and Mott insulators: Hubbard U instead of Stoner I, Phys. Rev. B, 44 (1991) 943. https://doi.org/10.1103/PhysRevB.44.943
Wang H, Konashi K, LDA + U study of Pu and PuO2 on ground state with spin–orbital coupling, J. Alloys Compounds, 533 (2012) 53–57. https://doi.org/10.1016/j.jallcom.2012.03.117
DOI: https://doi.org/10.15826/elmattech.2023.2.017
Copyright (c) 2023 Galashev Y. Alexander, Ksenia A. Abramova, Alexey S. Vorob'ev, Oksana R. Rakhmanova

This work is licensed under a Creative Commons Attribution 4.0 International License.