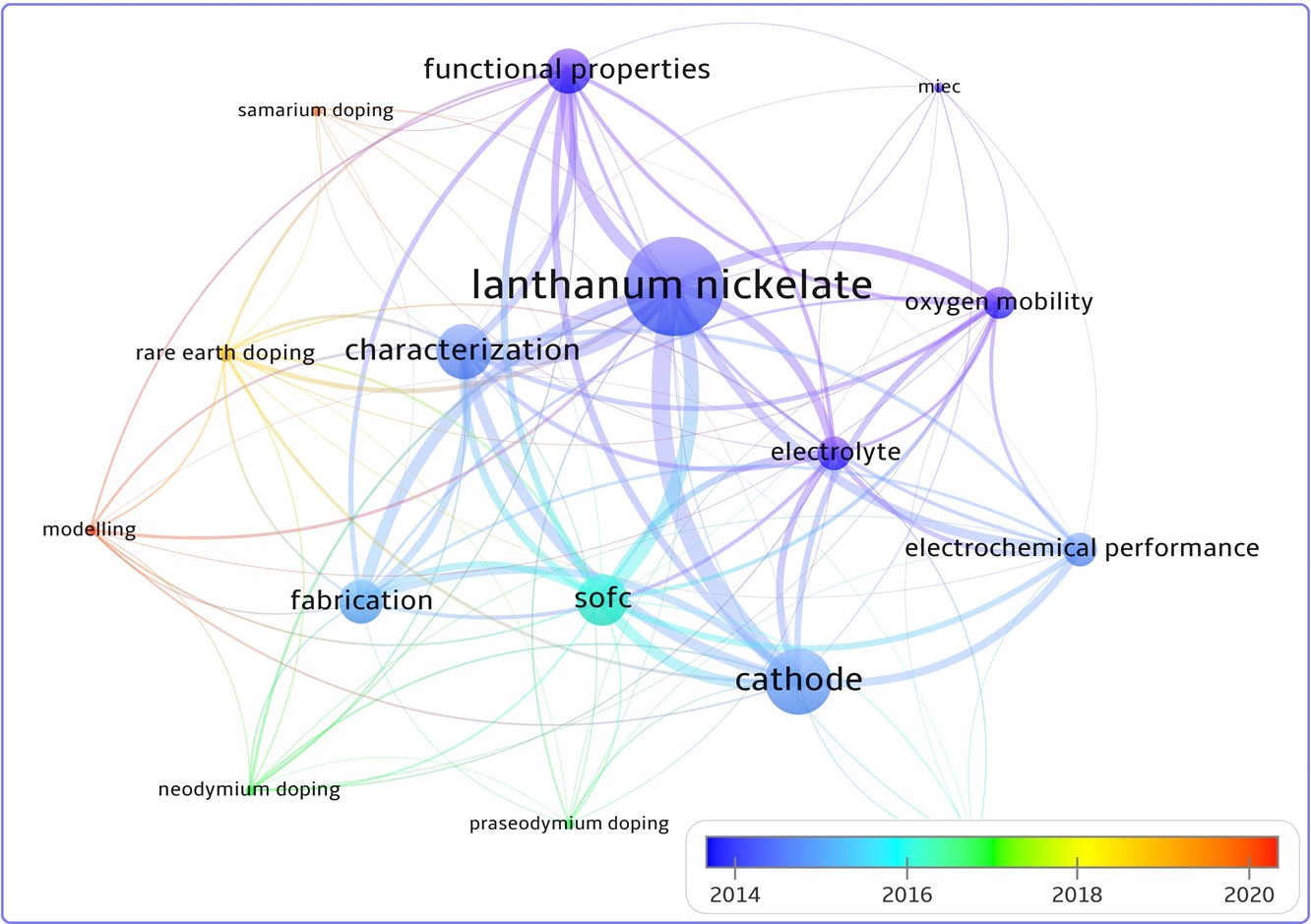
Short review on recent studies and prospects of application of rare-earth-doped La2NiO4+δ as air electrodes for solid-oxide electrochemical cells
Abstract
Solid solutions based on the rare earth substituted lanthanum nickelate La2NiO4+δ are considered as promising air electrode materials for electrochemical applications. The present focus review summarizes recently published papers dealing with synthesis methods and investigations of the crystal structure, physicochemical properties, oxygen diffusion and electrochemical activity of La2–xLnxNiO4+δ (Ln = Pr, Nd, Sm, Eu, Gd) electrode materials. It highlights the application advantages and drawbacks of the Ln-substituted La2NiO4+δ for solid oxide fuel and electrolysis cells and compared to the non-substituted La2NiO4+δ.
Keywords
Full Text:
PDFReferences
Nirala G, Yadav D, Upadhyay S, Ruddlesden-Popper phase A2BO4 oxides: Recent studies on structure, electrical, dielectric, and optical properties, J. Adv. Ceram., 9 (2020) 129–148. https://doi.org/10.1007/s40145-020-0365-x
Morales-Zapata MA, Larrea A, Laguna-Bercero MA, Lanthanide nickelates for their application on Solid Oxide Cells, Electrochim. Acta, 444 (2023) 141970. https://doi.org/10.1016/j.electacta.2023.141970
Ding P, Li W, Zhao H, et al., Review on Ruddlesden–Popper perovskites as cathode for solid oxide fuel cells, J. Phys. Mater., 4 (2021) 022002. https://doi.org/10.1088/2515-7639/abe392
Yatoo MA, Habib F, Malik AH, et al., Solid-oxide fuel cells: A critical review of materials for cell components, MRS Comm., 13 (2023) 378–384. https://doi.org/10.1557/s43579-023-00371-0
Wang Q, Fan H, Xiao Y, Zhang Y, Applications and recent advances of rare earth in solid oxide fuel cells, J Rare Earths, 40 (2022) 1668–1681. https://doi.org/10.1016/j.jre.2021.09.003
Manthiram A, Kim J-H, Kim YN, Lee K-T, Crystal chemistry and properties of mixed ionic-electronic conductors, J. Electroceram., 27 (2011) 93–107. https://doi.org/10.1007/s10832-011-9635-x
Antonova EP, Osinkin DA, Bogdanovich NM, et al., Electrochemical performance of Ln2NiO4+δ (Ln – La, Nd, Pr) and Sr2Fe1.5Mo0.5O6−δ oxide electrodes in contact with apatite-type La10(SiO6)4O3 electrolyte, Solid State Ion., 329 (2019) 82–89. https://doi.org/10.1016/j.ssi.2018.11.019
Antonova EP, Kolchugin AA, Pikalova EYu, et al., Development of electrochemically active electrodes for BaCe0.89Gd0.1Cu0.01O3−δ proton conducting electrolyte, Solid State Ion., 306 (2017) 55–61. https://doi.org/10.1016/j.ssi.2017.02.001
Sadykov VA, Sadovskaya EM, Eremeev NF, et al., Oxygen mobility in the materials for Solid Oxide Fuel cells and catalytic membranes (Review), Russ. J. Electrochem., 55 (2019) 701–718. https://doi.org/10.1134/S1023193519080147
Yang S, Liu G, Lee Y-L, et al., A systematic ab initio study of vacancy formation energy, diffusivity, and ionic conductivity of Ln2NiO4+δ (Ln=La, Nd, Pr), J. Power Sources, 576 (2023) 233200. https://doi.org/10.1016/j.jpowsour.2023.233200
Lee D, Lee H, Controlling oxygen mobility in Ruddlesden–Popper oxides, Materials, 10 (2017) 368. https://doi.org/10.3390/ma10040368
Chroneos A, Vovk RV, Goulatis IL, Goulatis LI, Oxygen transport in perovskite and related oxides: A brief review, J. Alloys Compd., 494 (2010) 190–195. https://doi.org/10.1016/j.jallcom.2010.01.071
Istomin SYa, Lyskov NV, Mazo GN, Antipov EV, Electrode materials based on complex d-metal oxides for symmetrical solid oxide fuel cells, Russ. Chem. Rev., 90 (2021) 644–676. https://doi.org/10.1070/RCR4979
Klyndyuk AI, Chizhova EA, Kharytonau DS, Medvedev DA, Layered oxygen-deficient double perovskites as promising cathode materials for solid oxide fuel cells, Materials, 15 (2021) 141. https://doi.org/10.3390/ma15010141
Tarutin AP, Lyagaeva JG, Medvedev DA, et al., Recent advances in layered Ln2NiO4+δ nickelates: Fundamentals and prospects of their applications in protonic ceramic fuel and electrolysis cells, J. Mater. Chem. A, 9 (2021) 154–195. https://doi.org/10.1039/d0ta08132a
Nikonov AV, Kuterbekov KA, Bekmyrza KZh, Pavzderin NB, A brief review of conductivity and thermal expansion of perovskite-related oxides for SOFC cathode, Euras. J. Phys. Funct. Mater., 2 (2018) 274–292. https://doi.org/10.29317/ejpfm.2018020309
Koval’chuk AN, Kuz’min AV, Osinkin DA, et al., Single SOFC with supporting Ni-YSZ anode, bilayer YSZ/GDC film electrolyte, and La2NiO4+δ cathode, Russ. J. Electrochem., 54 (2018) 541–546. https://doi.org/10.1134/S1023193518060101
Pikalova EYu, Bogdanovich NM, Kolchugin AA, et al., Electrical and electrochemical properties of La2NiO4+δ-based cathodes in contact with Ce0.8Sm0.2O2−δ electrolyte, Procedia Eng., 98 (2014) 105–110. https://doi.org/10.1016/j.proeng.2014.12.495
Antonova EP, Khodimchuk AV, Usov GR, et al., EIS analysis of electrode kinetics for La2NiO4+δ cathode in contact with Ce0.8Sm0.2O1.9 electrolyte: from DRT analysis to physical model of the electrochemical process, J. Solid State Electrochem., 23 (2019) 1279–1287. https://doi.org/10.1007/s10008-019-04224-6
Antonova EP, Stroeva AYu, Tropin ES, Electrode performance of La2NiO4+δ cathodes in contact with La0.9Sr0.1ScO3−δ proton-conducting oxide, J. Solid State Electrochem., 24 (2020) 1447–1451. https://doi.org/10.1007/s10008-020-04535-z
Antonova EP, Khodimchuk AV, Tropin ES, et al., Influence of modifying additives on electrochemical performance of La2NiO4+δ - based oxygen electrodes, Solid State Ion., 346 (2020) 115215. https://doi.org/10.1016/j.ssi.2019.115215
Tarutin AP, Lyagaeva JG, Farlenkov AS, et al., Cu-substituted La2NiO4+δ as oxygen electrodes for protonic ceramic electrochemical cells, Ceram. Int., 45 (2019) 16105–16112. https://doi.org/10.1016/j.ceramint.2019.05.127
Kovrova AI, Gorelov VP, Kuzmin AV, et al., Influence of Ce0.8R0.2O2–a (R = Y, Sm, Tb) submicron barrier layers at the La2NiO4+δ/YSZ boundary on the electrochemical performance of a cathode, J. Solid State Electrochem., 25 (2021) 1789–1796. https://doi.org/10.1007/s10008-021-04942-w
Cherepanov VA, Gilev AR, Kiselev EA, Electrotransport in the La2NiO4-based solid solutions, Pure Appl. Chem., 91 (2019) 911–922. https://doi.org/10.1515/pac-2018-1001
Kol’chugin AA, Pikalova EYu, Bogdanovich NM, et al., Electrochemical properties of doped lantanum–nickelate-based electrodes, Russ. J. Electrochem., 53 (2017) 826–833. https://doi.org/10.1134/S1023193517080110
Pikalova E, Sadykov V, Sadovskaya E, et al., Correlation between structural and transport properties of Ca-doped La nickelates and their electrochemical performance, Crystals, 11 (2021) 297. https://doi.org/10.3390/cryst11030297
Xu S, Jacobs R, Morgan D, Factors controlling oxygen interstitial diffusion in the Ruddlesden–Popper oxide La2–xSrxNiO4+δ, Chem. Mater. 30 (2018) 7166–7177. https://doi.org/10.1021/acs.chemmater.8b03146
Gilev AR, Kiselev EA, Zakharov DM, Cherepanov VA, Effect of calcium and copper/iron co-doping on defect-induced properties of La2NiO4-based materials, J. Alloys Compd., 753 (2018) 491–501. https://doi.org/10.1016/j.jallcom.2018.04.178
Antonova EP, Khodimchuk AV, Tropin ES, et al., Influence of polarization on the electrochemical activity of La2–xCaxNiO4+δ electrodes in contact with Ce0.8Sm0.2O1.9 electrolyte, Int. J. Hydrogen Energy, 48 (2023) 22585–22593. https://doi.org/10.1016/j.ijhydene.2023.01.343
Sadykov VA, Sadovskaya EM, Pikalova EYu, et al., Transport features in layered nickelates: correlation between structure, oxygen diffusion, electrical and electrochemical properties, Ionics, 24 (2018) 1181–1193. https://doi.org/10.1007/s11581-017-2279-3
Türk H, Götsch T, Schmidt F, et al., Sr surface enrichment in solid oxide cells – approaching the limits of EDX analysis by multivariate statistical analysis and simulations, ChemCatChem, 14 (2022) e202200300. https://doi.org/10.1002/cctc.202200300
Wu X, Gu C, Cao J, et al., Investigations on electrochemical performance of La2NiO4+δ cathode material doped at A site for solid oxide fuel cells, Mater. Res. Express, 7 (2020) 065507. https://doi.org/10.1088/2053-1591/ab9c60
Gilev AR, Kiselev EA, Ozhiganov ME, Cherepanov VA, Factors governing surface exchange kinetics in undoped and strontium/iron co-substituted La2NiO4+δ, Int. J. Hydrogen Energy, 48 (2023) 22573–22584. https://doi.org/10.1016/j.ijhydene.2023.01.182
Pikalova E, Eremeev N, Sadovskaya E, et al., Influence of the substitution with rare earth elements on the properties of layered lanthanum nickelate – Part 1: Structure, oxygen transport and electrochemistry evaluation, Solid State Ion., 379 (2022) 115903. https://doi.org/10.1016/j.ssi.2022.115903
Pikalova E, Kolchugin A, Tsvinkinberg V, et al., Comprehensive study of functional properties and electrochemical performance of layered lanthanum nickelate substituted with rare-earth elements, J. Power Sources, 581 (2023) 233505. https://doi.org/10.1016/j.jpowsour.2023.233505
Li X, Benedek NA, Enhancement of ionic transport in complex oxides through soft lattice modes and epitaxial strain, Chem. Mater., 27 (2015) 2647–2652. https://doi.org/10.1021/acs.chemmater.5b00445
Gilev AR, Kiselev EA, Ozhiganov ME, Cherepanov VA, Polarization resistance of the Ruddlesden-Popper nickelates Lan+1NinO3n+1 (n = 1, 2, 3): Comparative analysis using the distribution of relaxation times method, Solid State Ion., 386 (2022) 116032. https://doi.org/10.1016/j.ssi.2022.116032
Flura A, Dru S, Nicollet C, et al., Chemical and structural changes in Ln2NiO4 (Ln=La, Pr or Nd) lanthanide nickelates as a function of oxygen partial pressure at high temperature, J. Solid State Chem., 228 (2015) 189–198. https://doi.org/10.1016/j.jssc.2015.04.029
Ruck K, Krabbes G, Vogel I, Structural and electrical properties of La2−xCaxNiO4+δ (0 ≤ x < 0.4) with regard to the oxygen content δ, Mat. Res. Bull., 34 (1999) 1689–1697. https://doi.org/10.1016/S0025-5408(99)00163-4
Jorgensen JD, Dabrowski B, Pei S, et al., Structure of the interstitial oxygen defect in La2NiO4+δ, Phys. Rev. B, 40 (1989) 2187–2199. https://doi.org/10.1103/PhysRevB.40.2187
Choi SR, Lee J-I, Park H, et al., Multiple perovskite layered lanthanum nickelate Ruddlesden-Popper systems as highly active bifunctional oxygen catalysts, Chem. Eng. J., 409 (2021) 128226. https://doi.org/10.1016/j.cej.2020.128226
Tsvinkinberg VA, Tolkacheva AS, Filonova EA, et al., Structure, thermal expansion and electrical conductivity of La2–xGdxNiO4+δ (0.0 ≤x≤ 0.6) cathode materials for SOFC applications, J. Alloys Compd., 853 (2021) 156728. https://doi.org/10.1016/j.jallcom.2020.156728
Amira S, Ferkhi M, Khaled A, et al., Carbon-based lanthanum nickelate material La2−x−yNdxPryNiO4+δ (x = 0, 0.3, and 0.5; y = 0 and 0.2) as a bifunctional electrocatalyst for oxygen reduction in alkaline media, Ionics, 25 (2019) 3809–3822. https://doi.org/10.1007/s11581-019-02963-0
Boehm E, Bassat J, Dordor P, et al., Oxygen diffusion and transport properties in non-stoichiometric Ln2−xNiO4+δ oxides, Solid State Ion., 176 (2005) 2717–2725. https://doi.org/10.1016/j.ssi.2005.06.033
Sharma RK, Khamidy NI, Bassat JM, Djurado E, La2−xPrxNiO4+δ-based efficient SOFC cathodes: Effect of microstructure, composition and architecture, ECS Trans., 78 (2017) 581–591. https://doi.org/10.1149/07801.0581ecst
Kolchugin AА, Pikalova EYu, Bogdanovich NM, et al., Structural, electrical and electrochemical properties of calcium-doped lanthanum nickelate, Solid State Ion., 288 (2016) 48–53. https://doi.org/10.1016/j.ssi.2016.01.035
Naumovich EN, Kharton VV, Atomic-scale insight into the oxygen ionic transport mechanisms in La2NiO4-based materials, J. Mol. Struct., 946 (2010) 57–64. https://doi.org/10.1016/j.theochem.2009.12.003
Minervini L, Grimes RW, Kilner JA, Sickafus KE, Oxygen migration in La2NiO4+δ, J. Mater. Chem., 10 (2000) 2349–2354. https://doi.org/10.1039/b004212i
Chroneos A, Parfitt D, Kilner JA, Grimes RW, Anisotropic oxygen diffusion in tetragonal La2NiO4+δ : molecular dynamics calculations, J. Mater. Chem., 20 (2010) 266–270. https://doi.org/10.1039/B917118E
Li X, Huan D, Shi N, et al., Defects evolution of Ca doped La2NiO4+δ and its impact on cathode performance in proton-conducting solid oxide fuel cells, Int. J. Hydrogen Energy, 45 (2020) 17736–17744. https://doi.org/10.1016/j.ijhydene.2020.04.150
Skinner S, Oxygen diffusion and surface exchange in La2−xSrxNiO4+δ, Solid State Ion., 135 (2000) 709–712. https://doi.org/10.1016/S0167-2738(00)00388-X
Tropin ES, Ananyev MV, Farlenkov AS, et al., Surface defect chemistry and oxygen exchange kinetics in La2−xCaxNiO4+δ, J. Solid State Chem., 262 (2018) 199–213. https://doi.org/10.1016/j.jssc.2018.03.020
Pikalova EYu, Kolchugin AA, Sadykov VA, et al., Structure, transport properties and electrochemical behavior of the layered lanthanide nickelates doped with calcium, Int. J. Hydrogen Energy, 43 (2018) 17373–17386. https://doi.org/10.1016/j.ijhydene.2018.07.115
Gauquelin N Impact of the structural anisotropy of La2NiO4+δ on high temperature surface modifications and diffusion of oxygen [Doctorale Science Theses] Rennes (France): Universite De Rennes; 2010. 226 p. http://publications.rwth-aachen.de/record/82802/files/3662.pdf
Bassat J, Anisotropic ionic transport properties in La2NiO4+δ single crystals, Solid State Ion., 167 (2004) 341–347. https://doi.org/10.1016/j.ssi.2003.12.012
Matsuda M, Hashimoto M, Matsunaga C, et al., Electrophoretic fabrication of a-b plane oriented La2NiO4 cathode onto electrolyte in strong magnetic field for low-temperature operating solid oxide fuel cell, J. Eur. Ceram. Soc., 36 (2016) 4077–4082. https://doi.org/10.1016/j.jeurceramsoc.2016.06.043
Shin J, Yang S, Ji H-I, et al., Low-temperature processing technique of Ruddlesden-Popper cathode for high-performance solid oxide fuel cells, J. Alloys Compd., 868 (2021) 159092. https://doi.org/10.1016/j.jallcom.2021.159092
Inprasit T, Limthongkul P, Wongkasemjit S, Sol-gel and solid-state synthesis and property study of La2−xSrxNiO4 (x ≤ 0.8), J. Electrochem. Soc., 157 (2010) B1726. https://doi.org/10.1149/1.3489262
Lyagaeva J, Medvedev D, Pikalova E, et al., A detailed analysis of thermal and chemical compatibility of cathode materials suitable for BaCe0.8Y0.2O3−δ and BaZr0.8Y0.2O3−δ proton electrolytes for solid oxide fuel cell application, Int. J. Hydrogen Energy, 42 (2017) 1715–1723. https://doi.org/10.1016/j.ijhydene.2016.07.248
Sameshima S, Kawaminami M, Hirata Y, Thermal expansion of rare-earth-doped ceria ceramics, J. Ceram. Soc. Japan, 110 (2002) 597–600. https://doi.org/10.2109/jcersj.110.597
Stevenson JW, Hasinska K, Canfield NL, Armstrong TR, Influence of cobalt and iron additions on the electrical and thermal properties of (La,Sr)(Ga,Mg)O3−δ, J. Electrochem. Soc., 147 (2000) 3213. https://doi.org/10.1149/1.1393885
Shaula A, Kharton V, Marques F, Ionic and electronic conductivities, stability and thermal expansion of La10−x(Si,Al)6O26±δ solid electrolytes, Solid State Ion., 177 (2006) 1725–1728. https://doi.org/10.1016/j.ssi.2005.11.023
Pikalova EYu, Kolchugin AA, The influence of the substituting element (M = Ca, Sr, Ba) in La1.7M0.3NiO4+δ on the electrochemical performance of the composite electrodes, Eur. Chem.-Tech. J., 18 (2016) 3. https://doi.org/10.18321/ectj386
Lesnichyova A, Stroeva A, Belyakov S, et al., Water uptake and transport properties of La1−xCaxScO3−α proton-conducting oxides, Materials, 12 (2019) 2219. https://doi.org/10.3390/ma12142219
Hernández AM, Mogni L, Caneiro A, La2NiO4+δ as cathode for SOFC: Reactivity study with YSZ and CGO electrolytes, Int. J. Hydrogen Energy, 35 (2010) 6031–6036. https://doi.org/10.1016/j.ijhydene.2009.12.077
Philippeau B, Mauvy F, Mazataud C, et al., Comparative study of electrochemical properties of mixed conducting Ln2NiO4+δ (Ln=La, Pr and Nd) and La0.6Sr0.4Fe0.8Co0.2O3−δ as SOFC cathodes associated to Ce0.9Gd0.1O2−δ, La0.8Sr0.2Ga0.8Mg0.2O3−δ and La9Sr1Si6O26.5 electrolytes, Solid State Ion., 249–250 (2013) 17–25. https://doi.org/10.1016/j.ssi.2013.06.009
Taş AC, Majewski PJ, Aldinger F, Chemical preparation of pure and strontium- and/or magnesium-doped lanthanum gallate powders, J. Am. Ceram. Soc., 83 (2000) 2954–2960. https://doi.org/10.1111/j.1151-2916.2000.tb01666.x
Maglia F, Anselmi-Tamburini U, Chiodelli G, et al., Electrical, structural, and microstructural characterization of nanometric La0.9Sr0.1Ga0.8Mg0.2O3−δ (LSGM) prepared by high-pressure spark plasma sintering, Solid State Ion., 180 (2009) 36–40. https://doi.org/10.1016/j.ssi.2008.10.005
Kesapragada SV, Bhaduri SB, Bhaduri S, Singh P, Densification of LSGM electrolytes using activated microwave sintering, J. Power Sources, 124 (2003) 499–504. https://doi.org/10.1016/j.jpowsour.2003.06.002
Song J, Ning D, Boukamp B, et al., Structure, electrical conductivity and oxygen transport properties of Ruddlesden–Popper phases Lnn+1NinO3n+1 (Ln = La, Pr and Nd; n = 1, 2 and 3), J. Mater. Chem. A, 8 (2020) 22206–22221. https://doi.org/10.1039/D0TA06731H
Lou Z, Dai N, Wang Z, et al., Preparation and electrochemical characterization of Ruddlesden–Popper oxide La4Ni3O10 cathode for IT-SOFCs by sol–gel method, J. Solid State Electrochem., 17 (2013) 2703–2709. https://doi.org/10.1007/s10008-013-2150-z
Pikalova E, Kolchugin A, Zakharchuk K, et al., Mixed ionic-electronic conductivity, phase stability and electrochemical activity of Gd-substituted La2NiO4+δ as oxygen electrode material for solid oxide fuel/electrolysis cells, Int. J. Hydrogen Energy, 46 (2021) 16932–16946. https://doi.org/10.1016/j.ijhydene.2021.03.007
Nakamura T, Yashiro K, Sato K, Mizusaki J, Electronic state of oxygen nonstoichiometric La2−xSrxNiO4+δ at high temperatures, Phys. Chem. Chem. Phys., 11 (2009) 3055. https://doi.org/10.1039/b823364k
Shaula AL, Naumovich EN, Viskup AP, et al., Oxygen transport in La2NiO4+δ: Assessment of surface limitations and multilayer membrane architectures, Solid State Ion., 180 (2009) 812–816. https://doi.org/10.1016/j.ssi.2009.01.005
Goodenough JB, Ramasesha S, Further evidence for the coexistence of localized and itinerant 3d electrons in La2NiO4, Mat. Res. Bull., 17 (1982) 383–390. https://doi.org/10.1016/0025-5408(82)90089-7
Goodenough JB, Bond-length mismatch in intergrowth structures, J. Less Common Met., 116 (1986) 83–93. https://doi.org/10.1016/0022-5088(86)90219-5
Bassat JM, Odier P, Loup JP, The Semiconductor-to-Metal Transition in Question in La2−xNiO4+δ (δ > 0 or δ < 0), J. Solid State Chem., 110 (1994) 124–135. https://doi.org/10.1006/jssc.1994.1146
Nishiyama S, Sakaguchi D, Hattori T, Electrical conduction and thermoelectricity of La2NiO4+δ and La2(Ni,Co)O4+δ, Solid State Comm., 94 (1995) 279–282. https://doi.org/10.1016/0038-1098(95)00062-3
Vashook VV, Tolochko SP, Yushkevich II, et al., Oxygen nonstoichiometry and electrical conductivity of the solid solutions La2−xSrxNiOy (0≤x≤0.5), Solid State Ion., 110 (1998) 245–253. https://doi.org/10.1016/S0167-2738(98)00134-9
Ananyev MV, Tropin ES, Eremin VA, et al., Oxygen isotope exchange in La2NiO4±δ, Phys. Chem. Chem. Phys., 18 (2016) 9102–9111. https://doi.org/10.1039/C5CP05984D
Zhao K, Wang Y-P, Chen M, et al., Electrochemical evaluation of La2NiO4+δ as a cathode material for intermediate temperature solid oxide fuel cells, Int. J. Hydrogen Energy, 39 (2014) 7120–7130. https://doi.org/10.1016/j.ijhydene.2014.02.106
Lee Y, Kim H, Electrochemical performance of La2NiO4+δ cathode for intermediate-temperature solid oxide fuel cells, Ceram. Int., 41 (2015) 5984–5991. https://doi.org/10.1016/j.ceramint.2015.01.037
Kim SJ, Kim KJ, Dayaghi AM, Choi GM, Polarization and stability of La2NiO4+δ in comparison with La0.6Sr0.4Co0.2Fe0.8O3−δ as air electrode of solid oxide electrolysis cell, Int. J. Hydrogen Energy, 41 (2016) 14498–14506. https://doi.org/10.1016/j.ijhydene.2016.05.284
Ogier T, Mauvy F, Bassat J-M, et al., Overstoichiometric oxides Ln2NiO4+δ (Ln = La, Pr or Nd) as oxygen anodic electrodes for solid oxide electrolysis application, Int. J. Hydrogen Energy, 40 (2015) 15885–15892. https://doi.org/10.1016/j.ijhydene.2015.09.107
Yoo Y-S, Choi M, Hwang J-H, et al., La2NiO4+δ as oxygen electrode in reversible solid oxide cells, Ceram. Int., 41 (2015)6448–6454. https://doi.org/10.1016/j.ceramint.2015.01.083
Filonova E, Pikalova E, Overview of approaches to increase the electrochemical activity of conventional perovskite air electrodes, Materials, 16 (2023) 4967. https://doi.org/10.3390/ma16144967
Vibhu V, Suchomel MR, Penin N, et al., Structural transformations of the La2−xPrxNiO4+δ system probed by high-resolution synchrotron and neutron powder diffraction, Dalton Trans, 48 (2019) 266–277. https://doi.org/10.1039/C8DT03524E
Sadykov VA, Pikalova EYu, Kolchugin AA, et al., Oxygen transport properties of Ca-doped Pr2NiO4, Solid State Ion., 317 (2018) 234–243. https://doi.org/10.1016/j.ssi.2018.01.035
Khamidy NI, Laurencin J, Ferreira Sanchez D, et al., Durability of nanostructured LaPrNiO4+δ electrode for solid oxide cells: Electrochemical, microstructural, and structural investigation, J. Power Sources, 450 (2020) 227724. https://doi.org/10.1016/j.jpowsour.2020.227724
Vibhu V, Flura A, Rougier A, et al., Electrochemical ageing study of mixed lanthanum/praseodymium nickelates La2−xPrxNiO4+δ as oxygen electrodes for solid oxide fuel or electrolysis cells, J. Energy Chem., 46 (2020) 62–70. https://doi.org/10.1016/j.jechem.2019.10.012
Kim GT, Jacobson AJ, Electrochemical characterization of La2−xPrxNiO4+x for application as cathodes in intermediate temperature SOFCs, MRS Proc., 972 (2006) 0972-AA11-04. https://doi.org/10.1557/PROC-0972-AA11-04
Zhao C, Zhou Q, Zhang T, et al., Preparation and electrochemical properties of La1.5Pr0.5NiO4 and La1.5Pr0.5Ni0.9Cu0.1O4 cathode materials for intermediate-temperature solid oxide fuel cells, Mat. Res. Bull., 113 (2019) 25–30. https://doi.org/10.1016/j.materresbull.2019.01.016
Nishimoto S, Takahashi S, Kameshima Y, et al., Properties of La2−xPrxNiO4 cathode for intermediate-temperature solid oxide fuel cells, J. Ceram. Soc. Japan, 119 (2011) 246–250. https://doi.org/10.2109/jcersj2.119.246
Sharma RK, Celikbilek O, Burriel M, et al., Electrochemical performance and chemical stability of architecturally designed La2−xPrxNiO4+δ IT-SOFC cathodes, ECS Trans., 72 (2016) 1–8. https://doi.org/10.1149/07233.0001ecst
Usenka A, Pankov V, Vibhu V, et al., Temperature programmed oxygen desorption and sorption processes on Pr2−xLaxNiO4+δ nickelates, ECS Trans., 91 (2019) 1341–1353. https://doi.org/10.1149/09101.1341ecst
Druce J, Kilner JA, Ishihara T, Relating Electrochemical performance measurements and surface analyses of Pr2−xLaxNiO4+δ electrode materials, ECS Trans., 57 (2013) 3269–3276. https://doi.org/10.1149/05701.3269ecst
Zhang Y, Wang W, Wang Y, et al., Role of Pr-vacancies and O-interstitials on the activity and stability of (Pr1−xLnx)2NiO4 (Ln = La, Nd, Pm, Sm, Gd, Tb, Dy, and Ho) towards oxygen reduction reactions: A DFT study, J. Electrochem. Soc., 168 (2021) 124508. https://doi.org/10.1149/1945-7111/ac4057
Vibhu V, Rougier A, Nicollet C, et al., La2−xPrxNiO4+δ as suitable cathodes for metal supported SOFCs, Solid State Ion., 78 (2015) 32–37. https://doi.org/10.1016/j.ssi.2015.05.005
Ishikawa H, Toyosumi Y, Ishikawa K, Structural phase transition of La2−xNdxNiO4+δ (0.0 ≤ x ≤ 2.0), J. Alloys Compd., 408–412 (2006) 1196–1199. https://doi.org/10.1016/j.jallcom.2004.12.143
Pikalov SM, Vedmid’ LB, Filonova EA, et al., High-temperature behavior of calcium substituted layered neodymium nickelates, J. Alloys Compd., 801 (2019) 558–567. https://doi.org/10.1016/j.jallcom.2019.05.349
Wan J, Goodenough J, Zhu J, Nd2−xLaxNiO4+δ, a mixed ionic/electronic conductor with interstitial oxygen, as a cathode material, Solid State Ion., 178 (2007) 281–286. https://doi.org/10.1016/j.ssi.2007.01.013
Amow G, Skinner SJ, Recent developments in Ruddlesden–Popper nickelate systems for solid oxide fuel cell cathodes, J. Solid State Electrochem., 10 (2006) 538–546. https://doi.org/10.1007/s10008-006-0127-x
Garali M, Kahlaoui M, Mohammed B, et al., Synthesis, characterization and electrochemical properties of La2−xEuxNiO4+δ Ruddlesden-Popper-type layered nickelates as cathode materials for SOFC applications, Int. J. Hydrogen Energy, 44 (2019) 11020–11032. https://doi.org/10.1016/j.ijhydene.2019.02.158
Sadykov VA, Sadovskaya EM, Filonova EA, et al., Oxide ionic transport features in Gd-doped La nickelates, Solid State Ion., 357 (2020) 115462. https://doi.org/10.1016/j.ssi.2020.115462
Wu CH, Shi YJ, Lu F, et al., Ruddlesden–Popper-type La1.5–xEuxPr0.5Ni0.9Cu0.1O4+δ as a potential cathode material for H-SOFCs, Phys. Solid State, 63 (2021) 775–784. https://doi.org/10.1134/S1063783421050218
Vegard L, Die konstitution der mischkristalle und die raumfllung der atome, Z Physik, 5 (1921) 17–26. https://doi.org/10.1007/BF01349680
Guseva EM, Ivanov RA, Pikalova EYu, Filonova EA, Modeling of crystal structure parameters in complex-oxide perovskite-like systems. In: Materials of the XIX Russian Conference «Physical Chemistry and Electrochemistry of molten and solid electrolytes»; 2023 Sep 17-21; Ekaterinburg, Russia. p. 188. http://conference.ihte2023.tilda.ws/sbornik
Choisnet J, Structure and bonding anisotropy in intergrowth oxides: A clue to the manifestation of bidimensionality in T-, T′-, and T*-type structures, J. Solid State Chem., 147 (1999) 379–389. https://doi.org/10.1006/jssc.1999.8381
Shannon RD, Revised effective ionic radii and systematic studies of interatomic distances in halides and chalcogenides, Acta Cryst. A, 32 (1976) 751–767. https://doi.org/10.1107/S0567739476001551
Goldschmidt VM, Die gesetze der krystallochemie, Naturwissenschaften, 14 (1926) 477–485. https://doi.org/10.1007/BF01507527
Ganguly P, Rao CNR, Crystal chemistry and magnetic properties of layered metal oxides possessing the K2NiF4 or related structures, J. Solid State Chem., 53 (1984) 193–216. https://doi.org/10.1016/0022-4596(84)90094-X
Vibhu V, Rougier A, Nicollet C, et al., La2−xPrxNiO4+δ as suitable cathodes for metal supported SOFCs, Solid State Ion., 278 (2015)32–37. https://doi.org/10.1016/j.ssi.2015.05.005
Guseva EM, Pikalova EYu, Tsvinkinberg VA, et al Crystal structure and physico-chemical properties of La2−xSmxNiO4+δ solid solutions. In: Abstracts of the XXXII Russian Youth Scientific Conference with International Participation Problems of Theoretical and Experimental Chemistry; 2022 Apr 19-22; Ekaterinburg, Russia. p. 162. http://hdl.handle.net/10995/117133
Kharton VV, Kovalevsky AV, Avdeev M, et al., Chemically induced expansion of La2NiO4+δ-based materials, Chem. Mater., 19 (2007) 2027–2033. https://doi.org/10.1021/cm070096x
Kharton VV, Yaremchenko AA, Shaula AL, et al., Transport properties and stability of Ni-containing mixed conductors with perovskite- and K2NiF4-type structure, J. Solid State Chem., 177 (2004) 26–37. https://doi.org/10.1016/S0022-4596(03)00261-5
Chaker H, Raies I, Chouket A, et al., Chemical and physical characterizations of the n = 1 Ruddlesden–Popper phases: Nd2−ySryNi1−xCoxO4±δ (y = 1 and 0.1 ≤ x ≤ 0.9), Ionics, 23 (2017) 2229–2240. https://doi.org/10.1007/s11581-017-2167-x
Shen Y, Zhao H, Liu X, Xu N, Preparation and electrical properties of Ca-doped La2NiO4+δ cathode materials for IT-SOFC, Phys. Chem. Chem. Phys., 12 (2010) 15124. https://doi.org/10.1039/c0cp00261e
Zakharchuk K, Kovalevsky A, Yaremchenko A, Characterization of Ruddlesden-Popper La2−xBaxNiO4±δ nickelates as potential electrocatalysts for solid oxide cells, Materials, 16 (2023) 1755. https://doi.org/10.3390/ma16041755
Bo G Oxygen reduction kinetics of La2−xSrxNiO4+δ electrodes for solid oxide fuel cells [Graduate Theses]. Morgantown (USA): West Virginia University; 2016. 58 p. https://researchrepository.wvu.edu/cgi/viewcontent.cgi?article=6759&context=etd
Aguadero A, Escudero MJ, Pérez M, et al., Effect of Sr content on the crystal structure and electrical properties of the system La2−xSrxNiO4+δ (0 ≤ x ≤ 1), Dalton Trans., 36 (2006) 4377–4383. https://doi.org/10.1039/B606316K
Hildenbrand N, Nammensma P, Blank DHA, et al., Influence of configuration and microstructure on performance of La2NiO4+δ intermediate-temperature solid oxide fuel cells cathodes, J. Power Sources, 238 (2013) 442–453. https://doi.org/10.1016/j.jpowsour.2013.03.192
Escudero MJ, Aguadero A, Alonso JA, Daza L, A kinetic study of oxygen reduction reaction on La2NiO4 cathodes by means of impedance spectroscopy, J. Electroanal. Chem., 611 (2007) 107–116. https://doi.org/10.1016/j.jelechem.2007.08.006
Nicollet C, Flura A, Vibhu V, et al., La2NiO4+δ infiltrated into gadolinium doped ceria as novel solid oxide fuel cell cathodes: Electrochemical performance and impedance modelling, J. Power Sources, 294 (2015) 473–482. https://doi.org/10.1016/j.jpowsour.2015.06.077
Kim GT, Wang S, Jacobson AJ, et al., Impedance studies of dense polycrystalline thin films of La2NiO4+δ, J. Mater. Chem., 17 (2007) 1316. https://doi.org/10.1039/b616101d
Ghamarinia M, Babaei A, Zamani C, Aslannejad H, Application of the distribution of relaxation time method in electrochemical analysis of the air electrodes in the SOFC/SOEC devices: A review, Chem. Eng. J. Adv., 15 (2023) 100503. https://doi.org/10.1016/j.ceja.2023.100503
Mauvy F, Lalanne C, Bassat J-M, et al., Electrode properties of Ln2NiO4+δ (Ln=La, Nd, Pr), J. Electrochem. Soc., 153 (2006) A1547. https://doi.org/10.1149/1.2207059
Adler S, Limitations of charge-transfer models for mixed-conducting oxygen electrodes, Solid State Ion., 135 (2000) 603–612. https://doi.org/10.1016/S0167-2738(00)00423-9
Lu Y, Kreller C, Adler SB, Measurement and modeling of the impedance characteristics of porous La1−xSrxCoO3−δ electrodes, J. Electrochem. Soc., 156 (2009) B513. https://doi.org/10.1149/1.3079337
Ascolani-Yael J, Montenegro-Hernández A, Garcés D, et al., The oxygen reduction reaction in solid oxide fuel cells: from kinetic parameters measurements to electrode design, J. Phys. Energy, 2 (2020) 042004. https://doi.org/10.1088/2515-7655/abb4ec
Chiu T-W, Lin M-X, Shih H-Y, et al., Preparation and performance of PrLaNiO4 and (La0.75Sr0.2Ba0.05)0.175Ce0.825O1.891 composite cathode material by solid state reaction for IT-SOFCs, Ceram. Int., 43 (2017) S700–S704. https://doi.org/10.1016/j.ceramint.2017.05.269
DOI: https://doi.org/10.15826/elmattech.2023.2.025
Copyright (c) 2023 Elena Yu. Pikalova, Ekaterina M. Guseva, Elena A. Filonova

This work is licensed under a Creative Commons Attribution 4.0 International License.